Abstract
Climate benefit assessments of bioenergy crops often focus on biogeochemical impacts, paying little if any attention to biogeophysical impacts. However, land conversions required for large-scale bioenergy crop production are substantial and may directly affect the climate by altering surface energy balance. In the US, such land conversions are likely to be met in part by converting Conservation Reserve Program (CRP) grassland to bioenergy crops. Here, we converted three 22 year old CRP smooth brome grass fields into no-till corn, switchgrass, or restored prairie bioenergy crops. We assessed the biogeophysical climate impact of the conversions using albedo changes relative to unconverted reference CRP grassland. The corn and perennial fields had higher annual albedo than the grassland they replacedâcausing cooling of the local climate. The cooling of the corn field occurred solely during the non-growing seasonâespecially when surfaces were snow-covered, whereas the cooling of the perennial fields was more prominent during the growing season. Compared to biogeochemical impacts with fossil fuel offsets for the same land conversions over eight years, the annual albedo-induced climate benefits add â¼35% and â¼78% to the annual biogeochemical benefits provided from the switchgrass and restored prairie fields, respectively, and offset â¼3.3% of the annual greenhouse gas (GHG) emissions from the corn field. We conclude that albedo-induced climate mitigation from conversion of CRP lands to perennial but not annual bioenergy crops can be substantial, and future climate impact assessments of bioenergy crops should include albedo changes in addition to GHG balances in order to better inform climate policies.
Export citation and abstract BibTeX RIS
1. Introduction
Land use and land management changes directly affect local to regional climate by altering surface energy balance through changes in albedo (Îα), sensible and latent heat energies, surface roughness and soil heat flux (biogeophysical processes) e.g. [1, 2], as well as greenhouse gas (GHG) balances through exchanges of CO2, CH4 and N2O between the land surface and the atmosphere (biogeochemical processes) e.g. [3]. Most climate impact assessments focus on the biogeochemical impacts, paying little if any attention to the biogeophysical impacts [4] due primarily to the difficulty of adequately reconciling biogeophysical impacts with global warming impact (GWI) metrics and challenges associated with the complex and non-linear effects of biogeophysical change on climate [5, 6]. However, because of their radiative nature, albedo-induced climate impacts can be expressed in GWI metrics for direct comparison with biogeochemical GWIs e.g. [7, 8]. Albedo-induced GWIs (GWIÎα ) from land use and land management changes have been increasingly studied [9â14], particularly in high-latitude forests with seasonal snow cover where the influence of albedo on regional and global climate is potentially higher than the influences of biogeochemical impacts [7, 15].
Although bioenergy crops can contribute to a sustainable energy future [16], large-scale bioenergy crop production requires extensive land use likely to be met in part by converting set-aside lands such as those in the USDA Conservation Reserve Program (CRP) to bioenergy production. Biogeochemical GWIs of such conversions have been modeled [17] and measured [18â20]. On the other hand, GWIÎα of such conversions are largely unknown, although model studies of converting annual to perennial bioenergy crops indicate that GWIÎα could be substantial. For example, Georgescu et al [10] estimated that the GWIÎα benefit from converting tilled corn (Zea mays L.)-soybean (Glycine max L.) fields to switchgrass (Panicum virgatum L.) bioenergy crops across the Central US was six-fold higher than the annual biogeochemical GWI benefit that arises from offsetting fossil fuel use. Caiazzo et al [12] estimated that the GWIÎα benefit for this conversion was 14-fold higher than the biogeochemical GWI benefit but negligible for converting uncultivated land to canola (Brassica napus L.). Thus, changes in albedo relative to changes in GHG exchanges could considerably influence the bioenergy crop's climate impact depending on prior land use and crop species. However, Georgescu et al [10] and Caiazzo et al [12] did not include albedo changes and consequent GWIÎα in the presence of snow, which could be substantial and potentially counteract the growing season climate benefits e.g. [13, 21].
Few studies also report measured albedo change following bioenergy conversion. For example, in-situ albedo measurements in Miller et al [21] and Eichelman et al [22] indicated local climate cooling in the US (central Illinois) and Canada (southern Ontario), respectively, from converting tilled corn-soybean fields to switchgrass bioenergy crops. Loarie et al [23], using satellite-derived albedo observations, reported regional climate cooling in the Brazilian cerrado from converting large-scale crop and pasture to sugarcane bioenergy production. However, satellite-derived albedo observations are acquired only every eight+ days, and their accuracy may be affected by clouds, and in locations with seasonal snow, by snow cover durations [24].
Here, we investigate albedo-induced climate impacts of converting 22 year old CRP grasslands dominated by smooth brome grass to no-till corn, switchgrass, or restored prairie (mixed native prairie species) [25] bioenergy cropping systems. We calculated albedo from in-situ, year-round 30 min solar radiation observations. We assessed the climate impacts of converting CRP grasslands to the bioenergy crops using albedo changes (Îα), shortwave radiative forcing (RFÎα ) and GWIÎα . We hypothesized that converting CRP smooth brome grass to perennial bioenergy crops (switchgrass or restored prairie) will result in increased surface albedo and thus cooling of the local climate, whereas converting to a no-till corn bioenergy crop will result in diminished albedo and thus warming of the local climate due to differences in phenology, canopy structure and management practices.
2. Materials and methods
2.1. Study sites
Study sites are located within the northeastern part of the US Midwest Corn Belt in southwest Michigan at the Great Lakes Bioenergy Research Center of the Kellogg Biological Station's Long-term Ecological Research site (42°N, 85°
W, 256 m asl) (figure 1). The region has a humid continental temperate climate. Mean annual air temperature is 10.2 °C and total annual precipitation averages 1005 mm with about half falling as snow (1981â2010) [26]. Total annual snowfall averages 1.3 m with three-quarters typically falling in DecemberâFebruary [26]. Soils are well-drained Typic Hapludalfs developed on glacial outwash [27] intermixed with loess [28].
Figure 1. Location of the study sites in southwest Michigan at the Kellogg Biological Station (KBS). The four component net radiometers (CNR1s) were placed at the center of the red circles. All fields had been planted to smooth brome grass under the USDA Conservation Reserve Program (CRP) grasslands in 1987. The CRP-Ref grassland (42°N, 85°
W, 246 m asl, 9 ha) was maintained in smooth brome grass to serve as a reference CRP grassland while the other three fields were converted to soybean in 2009 and then to no-till continuous corn (42°
N, 85°
W, 258 m asl, 17 ha), restored prairie (42°
N, 85°
W, 259 m asl, 11 ha), or switchgrass (42°
N, 85°
W, 262 m asl, 13 ha) from 2010 onwards. Basemap reproduced from Esri (2011). Basemap sources: ESRI, HERE, Garmin, USGS, Intermap, INCREMENT P, NRCAN, ESRI Japan, METI, ESRI China (Hong Kong), NOSTRA, © OpenStreetMap contributors, and the GIS User Community, https://www.arcgis.com/apps/mapviewer/index.html?webmap=8b3d38c0819547faa83f7b7aca80bd76.
Download figure:
Standard image High-resolution imageFour conventionally-tilled row-crop agricultural fields were set-aside in 1987 and planted to smooth brome grassâan introduced cool-season C3 grass of Eurasian originâfor enrollment in the CRP. Three of the four fields were converted to no-till soybean in 2009; and to either no-till continuous corn, switchgrass or mixed native prairie from 2010 onwards (figure 1). Corn was planted in early May and harvested around mid-October each year (table S1 (available online at stacks.iop.org/ERL/16/084059/mmedia)). Switchgrass and mixed prairie were planted in 2010 and harvested annually around November following autumn senescence from 2011 onwards. Corn and switchgrass were fertilized at â¼180 and â¼56 kg N haâ1 yrâ1, respectively. Restored prairie was not fertilized. The fourth field remained in smooth brome grass as a reference CRP grassland (CRP-Ref) and received no agronomic management. For details on land conversion and management see Abraha et al [29].
2.2. Incident and reflected solar radiation
Incident and surface-reflected solar radiation were measured at all sites using four component net radiometers (CNR1, Kipp & Zonen, Delft, The Netherlands) placed â¼1.5 m above average canopy height. The radiation sensors were inter-calibrated among sitesâfor incident solar radiation on cloudless summer days, and for reflected solar radiation on cloudless winter days when surfaces were uniformly covered by snowâassuming that measurements for the respective conditions were the same across all sites. Data were logged every 30 min using Campbell CR5000 dataloggers (CR5000, Campbell Scientific Inc. Logan, UT, USA). Surface albedo (α) was computed as a ratio of the 30 min reflected to incident solar radiation between an hour after sunrise and an hour before sunset from October 2013 to September 2018. Precipitation and snow depth were retrieved from a weather station located â¼4 km from the study sites (https://lter.kbs.msu.edu/datatables).
2.3. Shortwave radiative forcing
Change in surface albedo (Îα) from converting CRP-Ref grassland to corn, switchgrass or restored prairie bioenergy crops was computed as:

where αbio is albedo of the bioenergy crops, and αref is albedo of the CRP-Ref grassland. Shortwave radiative forcing at the top of the atmosphere () was then computed as [30]:

where n is number of days, SWin is the incident solar radiation on the surface, and Ta is the upward atmospheric transmittanceâassumed equivalent to downward atmospheric transmittance and computed as a ratio of incident solar radiation to solar radiation at the top of the atmosphere (see supplementary material). Shortwave radiative forcing was converted into global warming impact (GWIÎα ; kg CO2-eq mâ2 yrâ1) [6â8, 31]:
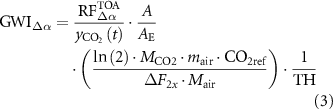
where A refers to the perturbed area (1 m2), AE to the earth's surface area (5.1 × 1014 m2), ÎF2x
to the radiative forcing per doubling of current CO2 concentration in the atmosphere (3.7 W mâ2), mair to mass of the atmosphere (5.148 × 1015 Mg), Mair and MCO2 to molecular weights of air (28.95 g molâ1) and CO2 (44.01 g molâ1), respectively, CO2ref to reference CO2 concentration in the atmosphere (389 ppmv), TH to time horizon (100 years) and to the airborne CO2 fraction that remains in the atmosphere at time t following a single pulse emissionâderived from multi-model impulse response function analysis [32]; see supplementary material.
2.4. Statistical analysis
Data were analyzed in the statistical software R [33]. Linear mixed model fits using the nlme package [34] were used to analyze albedo for the growing season (AprilâSeptember), non-growing season (OctoberâMarch) and the entire crop year (OctoberâSeptember), with crop type as fixed effects and years as random effects. Mean albedos among seasons/years and sites were compared using Tukey's HSD test with p-values adjusted using Bonferroni correction. Treatment effects were considered significant at p < 0.05. The model discriminates significant differences among years; however, since our experimental design lacked replication, significant differences among crop treatments could be due to treatment effects as well as underlying spatial heterogeneity among sites.
3. Results
3.1. Snowfall and snow depth
Long-term observations (1981â2010) (figure 2(a)) for the region show snowfalls could occur from October to April, with highest snowfall in DecemberâFebruary [26]. Total annual snowfall for our sites was higher in 2014 (2.2 m), similar in 2015 (1.2 m) but lower in 2016â2018 (0.8, 0.7 and 1.0 m, respectively) compared to the long-term mean of 1.3 m (figure 2(b)) [26]. Number of days with snow depth of >100 mm on the ground, indicating snowfall accumulation, for our sites (2014â2018) are shown in figure 2(c) with a rank order (days): 2014 (76), 2015 (46), 2018 (24), 2016 (14) and 2017 (13) along with the long-term mean (31) [26].
Figure 2. (a) Long-term mean (±1 standard error) monthly snowfall (1981â2010), (b) total annual snowfall (2014â2018 and long-term mean for 1981â2010) and (c) number of days with snow depth of >100 mm on the ground (2014â2018 and long-term mean for 1981â2010) at the Kellogg Biological Station [26].
Download figure:
Standard image High-resolution image3.2. Albedo
Changes in albedo from converting CRP-Ref grassland to corn showed similar average growing season albedo (αgs) but higher averages of non-growing season (αngs) and annual (αannual) albedos compared to those for the CRP-Ref grassland; conversion to switchgrass showed higher albedos than those for CRP-Ref grassland in all seasons; while conversion to restored prairie showed higher αgs but similar αngs and αannual compared to those for the CRP-Ref grassland (figures 3(a) and (b)).
Figure 3. (a) Average albedos for the growing season (αgs; AprilâSeptember), non-growing season (αngs; OctoberâMarch), and the entire year (αannual; OctoberâSeptember) of the corn, switchgrass, restored prairie and CRP-Ref (reference CRP smooth brome grass) fields from 2014 through 2018. Different letters indicate significant differences in average albedo between crop fields within a season (p < 0.05); and (b) average albedo differences (Îα) between the bioenergy cropping systems (corn (αC), switchgrass (αSw) or restored prairie (αPr)) and the CRP-Ref grassland (αref) for the growing season (Îαgs), non-growing season (Îαngs), and the entire year (Îαannual) from 2014 through 2018. Error bars are calculated as where SE is standard error, αbio is albedo of the bioenergy crop and αref is albedo of the reference crop. All fields were CRP grasslands, planted to smooth brome grass in 1987 and converted to soybean in 2009; and then to corn, switchgrass or restored prairie from 2010 onwards.
Download figure:
Standard image High-resolution imageAverage annual albedo during 2014â2018 for corn (0.30 ± 0.01; mean ± 1 standard error) was significantly higher than those for CRP-Ref grassland (0.26 ± 0.01; p< 0.001) and restored prairie (0.28 ± 0.01; p= 0.008), while αannual for switchgrass (0.29 ± 0.01) was not statistically distinguishable from αannual for corn or restored prairie, but was significantly higher than αannual for CRP-Ref grassland (p< 0.001; figure 3(a)). The αgs for switchgrass (0.21 ± 0.01) was significantly higher (p< 0.001) than αgs for all other crops in the study; and for restored prairie (0.20 ± 0.01) than αgs for CRP-Ref grassland and corn (0.19 ± 0.01; p< 0.001). The αngs for corn (0.42 ± 0.02) was significantly higher than αngs for all perennials (CRP-Ref grassland (0.35 ± 0.02; p< 0.001), restored prairie (0.36 ± 0.02; p< 0.001) and switchgrass (0.38 ± 0.02; p= 0.003)); and αngs for switchgrass was marginally higher (p< 0.046) than αn gs for CRP-Ref grassland. For all crops, αngs was â¼two-fold higher than αgs (p < 0.001). Annual and non-growing season cross-site albedos were highest in 2014 (p < 0.001) followed by 2015 (p < 0.001). Growing season cross-site albedos were similar among years except for significantly higher (p < 0.001) albedo in 2016 than in 2014, 2017 and 2018 (figure S1).
Monthly average albedos across years (αm) were significantly higher during the non-growing season (DecemberâMarch) than during the growing season, with the lowest αm in early autumn (SeptemberâOctober) for all cropping systems (figure 4). The highest αm for all perennials occurred in February and for corn in January. Corn had significantly higher αm than that for CRP-Ref grassland in AugustâSeptember and DecemberâFebruary, but significantly lower αm in MayâJuly, and statistically similar αm for all other months. Switchgrass had significantly higher αm than that for CRP-Ref grassland in AprilâOctober, but statistically similar albedos for all other months. Restored prairie showed the same statistical pattern as for switchgrass except for similar αm to that of CRP-Ref grassland in May.
Figure 4. Average monthly albedo (αm; 2014â2018) for corn, switchgrass, restored prairie and CRP-Ref (reference CRP smooth brome grass) cropping systems. Different letters indicate significant differences in αm between crop fields within a month (p < 0.05). Error bars represent ±1 standard error.
Download figure:
Standard image High-resolution image3.3. GWIÎα
Converting CRP-Ref grassland to corn, switchgrass or restored prairie fields caused, on average, cooling of the local climate (i.e. equivalent to net CO2 uptake) in all seasons, except for neutral effect during the growing season at the corn field. Converting CRP-Ref grasslands to corn, switchgrass and restored prairie resulted in average annual albedo-induced GWI (GWIÎα-annual; mean ± 1 se) of â0.31 ± 0.05, â0.52 ± 0.04 and â0.23 ± 0.04 MgCO2-eq haâ1 yrâ1; average growing season albedo-induced GWI (GWIÎα-gs) of â0.02 ± 0.05, â0.77 ± 0.05 and â0.28 ± 0.04 MgCO2-eq haâ1 gsâ1; and average non-growing season albedo-induced GWI (GWIÎα-ngs) of â0.61 ± 0.09, â0.27 ± 0.05, and â0.18 ± 0.07 MgCO2-eq haâ1 ngsâ1, respectively (figure 5).
Figure 5. Average albedo-induced global warming impacts (GWIÎα ) for conversions of CRP-Ref grassland (reference CRP smooth brome grass) fields to corn, switchgrass or restored prairie cropping systems during the growing season (GWIÎα-gs; AprilâSeptember), non-growing season (GWIÎα-ngs; OctoberâMarch), and the entire year (GWÎα-annual; OctoberâSeptember). GWIÎα for all conversions were calculated over a 100 year time horizon. Error bars represent ±1 standard error. Negative GWIÎα indicates a cooling effect equivalent to the uptake of CO2-eq.
Download figure:
Standard image High-resolution image4. Discussion
Converting CRP-Ref grassland to cornâcontrary to our hypothesisâas well as to switchgrass, or restored prairie bioenergy crops resulted, on average, in higher annual albedo (αannual) and cooling of the local climate. However, for corn, the cooling occurred solely during the non-growing seasonâespecially when surfaces were snow-coveredâwith no discernible albedo effect during the growing season. For the perennials, the cooling was more prominent during the growing season when surfaces were vegetation-covered. In general, albedo changes from converting CRP-Ref grassland to bioenergy cropping systemsâand the resulting GWIÎα âwere influenced by crop type, phenology, management, weather, and seasonality.
4.1. Albedo
Switchgrass and restored prairie fields had significantly higher αgs, whereas corn had statistically similar αgs compared to that of the CRP-Ref grassland they replaced (figures 3(a) and (b)). The αgs difference among the crop fields was likely due to leaf characteristics, crop morphology, as well as phenology and management practices such as timing of planting (for corn), green-up in spring (perennials), leaf area accumulation in summer, and eventual senescence and harvest. The perennial grasses usually green-up in April and close their canopies in May. The leaf area for switchgrass and restored prairie increases at a rate faster than that for CRP-Ref grassland, which explains their higher albedo during the growing season (figure 4). In contrast, corn is planted in early May and the ground remains relatively exposed. Hence, the corn field absorbs more solar radiation resulting in lower albedo than the perennial fields, at least until its canopy fully develops [35]. Corn had, however, significantly higher αm in AugustâSeptember than those for CRP-Ref grassland and restored prairie fields (figure 4).
Corn and switchgrass fields had significantly higher αngs, whereas the restored prairie field had statistically similar αngs compared to that of the CRP-Ref grassland they replaced (figures 3(a) and (b)). The αngs difference between the bioenergy crops and CRP-Ref grassland was likely due to over-winter canopy structure resulting from field management. The bioenergy crops were harvested at the end of each season and snowfalls on these fields create highly reflective white surfaces. In contrast, the CRP-Ref grassland was not harvested, with senesced plants standing throughout winter, at least partially masking snow cover and resulting in lower albedo than that of the harvested fields. For the unharvested CRP-Ref grassland to have similar albedo to those of the harvested fields, the snow had to accumulate deep enough to uniformly cover the plant stands. This likely depended on the amount and frequency of snowfall. Occasional, small snowfalls resulted in higher albedo of the harvested fields than that of the unharvested CRP-Ref grassland.
Corn also had significantly higher αngs than the harvested perennials. This was likely because the perennials remain unharvested for some time after corn harvest (table S1), which usually occurs before the onset of snow. Any snowfall after corn harvest but before harvest of the perennials results in higher albedo of the corn field than that of the perennial fields for reasons explained above. Even after all fields were harvested, corn may have higher albedo than the perennial fields due to differences in litter accumulation. The lower â¼10 cm of the perennial stem stalks were left behind following harvest. Some litter may also escape harvest (for example, when harvesting lodged grasses). In contrast, the corn fields were mowed and the stover partially harvested leaving less litter on the ground. This results in greater litter accumulation at the perennial fields than at the corn fields, which could potentially mask shallow snow layers resulting in lower albedo than at the corn fields. In agreement with this, Miller et al [21] reported lower albedo of switchgrass than that of corn when snow was present on the ground but overall similar αngs. The corn and perennial fields would likely have similar albedo once the snow depth is higher than any standing stem stalks and litter in the perennial fields.
Our observations that crop fields have higher winter albedo than grasslands agree with satellite-derived albedo observations by Zhao and Jackson [13], who found zonally averaged white-sky albedo around mid-January across North America over 45° Nâ60° N to be 0.57 for croplands and 0.50 for grasslands. Average albedo for January (2014â2018) at our sites ranged from 0.61 for corn to 0.51 for the CRP-Ref grassland (figure 4).
4.2. GWI
Converting CRP-Ref grassland to perennial and corn bioenergy crops caused local climate cooling on an annual scale, as indicated by negative GWIÎα-annual (figure 5). For conversion to perennials, the cooling was higher during the growing season (74% for switchgrass and 61% for restored prairie) than during the non-growing season, whereas for conversion to corn, the cooling solely occurred during the non-growing season (97%) with near-neutral warming during the growing season. Negative and positive Îα between corn and the CRP-Ref grassland fields early and late during the growing season, respectively, canceled out to yield near-neutral warming (figure 4). The non-growing season Îα between perennial bioenergy crops and CRP-Ref grassland was higher than that of the growing season, albeit with higher variation (figure 3(b)). However, shortwave radiative forcing per unit Îα (equation (2)) is higher during the growing season due to the higher incident solar radiation. In general, the perennials bring albedo-induced cooling in the summerâcounteracting warming, which could be vital in extreme hot weather [36], while the albedo-induced cooling provided by the corn field occurs in winter when it is already cold enough for snow to persist.
Converting CRP-Ref grassland to switchgrass, restored prairie and corn resulted in GWIÎα-annual of â0.52 ± 0.04, â0.23 ± 0.04 and â0.31 ± 0.05 Mg CO2-eq haâ1 yrâ1, respectively. The corresponding average annual biogeochemical GWIs that accounted for GHG fluxes, farming practices, agronomic inputs and fossil fuel offsets over eight years on the same fields were â1.5, â0.3 and 9.5 Mg CO2-eq haâ1 yrâ1, respectively (figure 6) [19, 37]. Comparing the GWIÎα-annual results of the present study with the biogeochemical GWI estimates of Abraha et al [19] indicates that the GWIÎα-annual benefit (cooling) provided by the switchgrass and restored prairie fields were â¼35% and â¼78% of the biogeochemical mitigation, respectively, whereas for corn field, the benefits offset â¼3.3% of the GHG emissions incurred over the eight-year period (figure 6). Not including the fossil fuel offsets, the GWIÎα-annual benefits reduced the annual net emissions (biogeochemical and biogeophysical) by 36%, 20%, and 2.2% for switchgrass, restored prairie and corn fields, respectively, over the eight-year period.
Figure 6. Annual global warming impacts (GWI; Mg CO2-eq haâ1 yrâ1) derived from albedo and greenhouse gas (GHG) exchanges (including net ecosystem C exchange adjusted for C in harvest (NEEadj), soil N2O and CH4 fluxes, farming inputs and fossil fuel offset credit for ethanol production) [19, 37] of corn, switchgrass and restored prairie bioenergy cropping systems converted from CRP-Ref grassland (reference CRP smooth brome grass). The GWIs induced by soil CH4 fluxes are too small to be visible in the stacked bar charts. Albedo-induced GWI was computed over a 100 year horizon using 5 year albedo observations (2014â2018) while GHG-induced GWIs were computed over an 8 year period (2009â2016) [19, 37]. All GWIs were computed using field observations with reference to unconverted CRP-Ref grassland. Negative GWI below the broken horizontal line indicates a cooling effect equivalent to the uptake of CO2-eq.
Download figure:
Standard image High-resolution imageOur study suggests that the â¼5.8 Mha CRP lands that have been converted to annual cropsâprimarily cornâsince 2007 in the US (USDA-FSA, 2020) had GWIÎα benefit of â¼â1.8 Mt CO2-eq yrâ1, assuming all the CRP lands were in smooth brome grass with similar climate and snowfall. If the â¼5.8 Mha CRP lands had been converted to switchgrass or restored prairie bioenergy crops instead of corn, the GWIÎα-annual benefit would have been â¼â3.0 Mt CO2-eq yrâ1 (almost two-fold higher than that of the corn field) or â¼â1.3 Mt CO2-eq yrâ1 (greater than two thirds of that of the corn field), respectively. In comparison, the biogeochemical GWI from same land conversions suggest an emission of â¼55.1 Mt CO2-eq yrâ1 for corn; and uptake of â¼â8.6 and â¼â1.7 Mt CO2-eq yrâ1 for switchgrass and restored prairie fields, respectively, for the eight years following conversion [19, 37]. It should be noted that CRP conversion to agricultural production results in very high biogeochemical emissions in the initial years of conversion, which decline over time and may eventually switch to GHG uptake as in the case of the perennials [19, 38]. Hence, following such conversions, biogeochemical GWIs show large inter-annual variations and may change direction from net GHG emission to net uptake over the lifetime of the crop while GWIÎα tend to be relatively constant and unidirectional.
Biogeochemical GWIs have highlighted climate benefit potentials of perennial bioenergy crops over annual cropping systemsâmainly corn [16, 18, 19, 39]. Converting tilled corn-soybean rotations to switchgrass and restored prairie bioenergy crops provided biogeochemical GWI benefits of â3.7 ± 0.7 and â4.6 ± 0.7 Mg CO2-eq haâ1 yrâ1, respectively, whereas converting to no-till continuous corn bioenergy crop was near-neutral (â0.2 ± 0.6 Mg CO2-eq haâ1 yrâ1) over eight years [19, 37]. Our present study suggests that if the CRP-Ref grasslands were converted to switchgrass instead of corn, the GWIÎα benefits, on average, would provide an additional â0.22 ± 0.06 Mg CO2-eq haâ1 yrâ1 savings, while conversion to restored prairie instead of corn would be near-neutral (0.08 ± 0.06 Mg CO2-eq haâ1 yrâ1). This suggests that converting no-till corn to switchgrass or restored prairie fields results in GWIÎα-annual of â0.22 ± 0.06 or 0.08 ± 0.06 Mg CO2-eq haâ1 yrâ1, respectively. However, the conversions to switchgrass and restored prairie fields indicated cooling during the growing season (GWIÎα-gs; â0.75 and â0.26 Mg CO2-eq haâ1 gsâ1, respectively) but warming during the non-growing season (GWIÎα-ngs; 0.31 and 0.41 Mg CO2-eq haâ1 ngsâ1, respectively). For corn to switchgrass conversions, Caiazzo et al [12] and Georgescu et al [10] reported GWIÎα-gs of â0.52 and â0.68 Mg CO2-eq haâ1 gsâ1, respectivelyâsimilar to our GWIÎα-gs of â0.75 Mg CO2-eq haâ1 gsâ1. Miller et al [21] also reported, for corn to switchgrass conversions, a warming in winterâin agreement with oursâalthough exact figures were not provided. In general, our findings of albedo-induced cooling benefit and near-neutral GWIÎα-annual from converting no-till corn bioenergy crop to switchgrass and restored prairie bioenergy crops, respectively, combined with the relatively higher biogeochemical climate benefits due to same conversions indicate greater climate benefits of perennials over the corn bioenergy crop.
Biogeochemical impacts also indicate that climate benefits are substantially higher when corn bioenergy croplands rather than CRP grasslands are converted to perennial bioenergy crops [19, 38]. Our findings suggest that converting CRP-Ref grasslands rather than existing corn bioenergy croplands to either switchgrass or restored prairie bioenergy crop results in GWIÎα-annual benefits of â¼â0.3 Mg CO2-eq haâ1 yrâ1âalthough almost all the benefits occur during the non-growing season. However, the biogeochemical GWI of converting CRP-Ref grasslands rather than existing corn bioenergy croplands to switchgrass and restored prairie bioenergy crops suggest GHG emissions of 2.2 and 4.3 Mg CO2-eq haâ1 yrâ1, respectively, over eight years [19, 37]. These reinforce the notion that climate benefits are higher when perennial bioenergy crops replace corn bioenergy croplands rather than conservation lands. It also demonstrates the overall higher climate benefits of perennial bioenergy crops (e.g. switchgrass and restored prairie) than that for corn e.g. [16, 18, 19].
A few additional caveats warrant mention: (a) Our results apply to settings with seasonal snow cover and would differ markedly if there were little or no snow accumulation; (b) albedo-induced GWIs considered in this study are for mature perennial stands, and may differ from albedo during conversion, planting and establishment of perennial fields; (c) GWIÎα in this study is for 100 year horizon, which assumes fields will remain unconverted for that long and shorter (e.g. 20 year) time horizons may enhance the cooling impact proportionately; and (d) the CRP has been discouraging planting of smooth brome grass in recent years in favor of diverse native grassland species that enhance wildlife habitat, although in humid temperate climates other grassland assemblages that might be used instead of smooth brome grass may have similar albedo as long as they are not harvested.
5. Conclusions
Conversion of CRP smooth brome grass fields to corn, switchgrass or restored prairie bioenergy crops resulted in higher annual albedos and resultant climate benefits. Climate benefits of converting CRP grassland to corn were solely in winter, especially when snow was present, whereas the benefits of converting to perennial cropping systems were pronounced in summer when surfaces were vegetation covered. Annual albedo-induced climate benefits added â¼35% and â¼78% of the annual net GHG mitigation with fossil fuel offset over eight years for conversions to switchgrass and restored prairie fields, respectively; and offset â¼3.3% of the annual net GHG emissions with fossil fuel offset for conversion to corn field. Climate impacts of bioenergy crops, particularly those involving CRP land use conversions, should account for albedo changes in addition to GHG balance to better inform climate policies.
Acknowledgments
We thank S Bohm, K Kahmark, J Simmons, Y-J Su, S VanderWulp, B Wilke, T Zenone and many others for field and data related assistance, and FC Alves of the MSU statistical consultancy center for help with statistical analysis. We also thank the two anonymous referees for helpful comments. Support for this research was provided by the Great Lakes Bioenergy Research Center, U.S. Department of Energy, Office of Science, Office of Biological and Environmental Research (Award DE-SC0018409), by the National Science Foundation Long-term Ecological Research Program (DEB 1832042) and USDA Long-term Agroecosystem Research Program at the Kellogg Biological Station, and by Michigan State University AgBioResearch.
Data availability statement
The data that support the findings of this study are available upon reasonable request from the authors.