Abstract
Interest in perchlorate as environmental pollutant has increased since 1997, when high concentrations have been found in the waters of the Colorado River, USA. Perchlorate is very persistent in nature and it is slowly degraded. Although harmful effects of large doses of perchlorate on thyroid function have been proven, the environmental effects are still unclear. The primary objective of the present review is to collect prevailing data of perchlorate exposure and to discuss its impact on human health. The results show that more than 50% of reviewed works found significant associations of perchlorate exposure and human health. This review consists of the following sections: general information of perchlorate sources, its properties and determination methods, role and sources in human body including food and water intake, overview of the scientific literature on the research on the effect of perchlorate on human health from 2010 to 2020. Finally, conclusions and recommendations on future perchlorate studies concerning human exposure are presented.
Introduction
Perchlorate has been identified as an environmental pollutant. Interest in perchlorate as potential substance affecting human health has increased since 1997, when large amounts of them have been detected in water samples from western states of the USA [1]. Sources of perchlorate in the environment may include those related to human activity and natural formation. Naturally occurring perchlorate were found in samples of Chilean nitrate, used on a large scale in agriculture as a fertilizer, probably also formed during reactions occurring in the upper regions of atmosphere. The sources related to human activity should involve applications in military purposes, space exploration, fireworks and other industries [2]. In the past years perchlorate was used as a hyperthyroidism treating agent, although it was withdrawn from medical usage due to its grave adverse effects [3]. Perchlorate has been classified as goitrogen (i.e., substance inhibiting iodine uptake by thyroid cells), which disturbs the metabolism of thyroid hormones and consequently can lead to serious health issues [4], [5]. Many studies were conducted to examine the effects of perchlorate on thyroid activity, while influence on other tissues and organs is still unknown. There are few papers describing the effect of perchlorate depending on the route of administration other than oral (inhalation, transdermal) and on other tissues and systems of the human body [6], [7]. Although the results of animal studies are available (mainly in rats) and significantly broaden the knowledge of perchlorate mode of action, their findings certainly require confirmation in humans [8]. Epidemiological research was mainly conducted in the US, partly also in China, but so far in other regions of the world have been carried out on a very small scale [8], [9]. The review of perchlorate toxicity in humans was published in 2010 in which the authors presented the outcomes of population exposure studies, multiple regression analyses of sodium-iodine symporter (NIS) inhibitors in urine and thyroid hormone concentration. Some of the occupational studies were included and the impact of gestational perchlorate exposure of newborn thyroid function were investigated. Conclusion of the aforementioned review indicates that there is no strong evidence between abnormal thyroid function and environmental perchlorate exposure, but there are some weaknesses of this study. Reviewed works were mainly conducted in the US and very few were from different geographical regions, such as East Asia or Europe. The investigated outcomes were focused directly on thyroid hormones or TSH levels and ignored many other serious health effects [10]. In the past decade many of new facts about perchlorate toxicity, involving non-thyroidal outcomes were acknowledged. A new mechanisms of perchlorate impact on NIS were proposed [11], so a novel approach on environmental perchlorate toxicity was taken in our review. The review focuses on the period of 2010–2020 to investigate into the most recent directions of perchlorate studies.
In this review we present the most actual knowledge of perchlorate chemistry, its environmental occurrence and human toxicity. The new data about perchlorate occurrence and health effect still reveals and there is essential need to re-evaluate periodically the state of knowledge on these compounds [12]. The main purpose of this work is to discuss findings of the human population studies from the peer-reviewed literature published in last 10 years which assessed potential risk of exposure and health effects. We also present the chemistry and some routes of exposure in order to facilitate the environmental perchlorate pollution. This review seeks to indicate the gaps of knowledge on perchlorate and attempts to outline new directions of prospective perchlorate studies.
Sources in the environment
Anthropogenic
Perchlorate was described for the first time by Count Friedrich von Stadion in the work “Von den Verbindungen des Chlorine mit dem Sauerstoff” in 1816 [13]. Significant increase in worldwide production of perchlorate was observed during World War II. In 1940 global production of ammonium perchlorate was about 2,000 tons per year, then in 1945 it was approximately 20,000 tons [14]. In this period perchlorate were mainly used for the production of explosives for war industry. After 1945, the production of perchlorate continued to grow especially for military applications, as their interesting properties were discovered as a valuable component of rocket fuel [15]. In conjunction with the current use of perchlorate in military installations, data on production sites and quantities of perchlorate production are only partially shown publicized. Thus, information on the annual production of perchlorate are inherently incomplete and consequently far from being actual. It is estimated that the annual global production of perchlorate is about several hundred thousand tons and the largest production plants are placed in the USA, France, Germany, Italy, China and Brazil [16], [17].
Anthropogenic sources include the import and use of Chilean nitrate as a fertilizer exploited from deposits in the Atacama desert in Chile. The fertilizer produced on the basis of Chilean nitrate contains approximately 98% NaNO3 and, according to various studies, from 0.05 to 0.4% ClO4− [18]. In the 1830–1980 period, the estimated volume of imports of Chilean saltpeter ore to the USA and Europe amounted to approximately 23 million tons in terms of nitrogen, with the highest value being reported in 1930s [19]. In US commercial production of sodium nitrate began in 1915 and in the following years domestic production increased its share in total US nitrate consumption. In 1994 over 11 million tons of sodium nitrate were used in US agriculture and only approximately 30,000 tons were from imported Chilean nitrate [2]. According to United Nations Trade Statistics Branch the volume of sodium nitrate import from Chile was about 19,000 tons in 2018 [20]. A material evidence of increasing man-made perchlorate contamination has been disclosed in shallow ice core in Thienshan, East Asia, where concentration of ClO4− was still increasing during 1980s’ to 2004 [21].
Natural
The largest known perchlorate reservoir in the world are the aforementioned Chilean saltpeter deposits. The presence of natural perchlorate has also been demonstrated in snow samples from Arctic, waters of the Great Lakes (USA and Canada), soil samples from Death Valley (USA) and Dry Valley (Antarctica) [1], [22], [23], [24], [25]. In 2015, the results of determination of perchlorate content in surface and underground waters as well as soil samples from semi-arid areas and deserts (Atacama Desert in Chile, Mojave Desert in the USA, deserts in western regions of Africa), showed the presence of ClO4− in range from 10−1 to 106 μg/kg [26]. The mechanism of formation of perchlorate in nature is not fully understood. Several paths of a possible perchlorate natural formation have been proposed [2], [26], [27], [28], [29], [30], [31], mainly in photochemical processes, with the participation of chloride ions and ozone in the troposphere and stratosphere [32]. The theory indicating the possibility of perchlorate formation during atmospheric discharges [2], [33] has not been explicitly confirmed [16]. It was also shown that the isotopic composition of perchlorate of natural origin (e.g., originating from Chilean nitrate) is different from those made artificially, which facilitates identification and determination of the source in the environment [34], [35].
Use of perchlorate
Some historical and current applications of perchlorate and perchloric acid are presented in Table 1.
Compound (group of compounds) | Product or process | Role |
---|---|---|
Salts of perchloric acid | Detonators | Oxidizer |
Matches | ||
Pyrotechnics | ||
Smoke candles | ||
Signal flares | ||
Explosives | ||
Fireworks | ||
Flash powder | ||
Airbags | ||
Rocket fuels | Propellant | |
Catapult seats | ||
Cells and batteries containing lithium | Electrolyte | |
Synthesis of ammonia | The component of the reaction mixture (reaction catalyst) | |
Metallurgy | Flux ingredient | |
Paint industry | Drying substance | |
PVC production | Additive increasing fire resistance | |
Perchloric acid | Gravimetric or precipitometric determination of potassium | The precipitating agent |
Tanning skins | Chromium extraction | |
Chemical and pharmaceutical industry | Oxidizer | |
Metallurgy | Extraction of rare Earth metals | |
Cellulose acetate production | Cellulose esterification | |
Titration in anhydrous environment | The strongest known acid in anhydrous acetic acid | |
Mineralization | The mineralizing agent (often a mixture of nitric acid (V) and sulfuric acid VI)) | |
Synthesis of ammonium chlorate (VI) | Starting material for synthesis |
Chemistry of perchlorate
Physical and chemical properties
Owing to the fact that perchlorate was discovered in 19th century, its chemistry seems to be well known [14], [37]. In this review article we gather the most important data on perchlorate properties in order to better understand its impact on human health. ClO4− is a simple inorganic anion with strong oxidizing properties. Perchloric acid is one of the strongest acids known and it is completely dissociated in water [38]. It has the structure of a tetrahedron composed of four oxygen atoms and one centrally located chlorine atom [14]. The average length of Cl-O bonds was determined experimentally by X-ray crystallography at 1.42 Å [39], [40]. It owes its strongly oxidizing character to the chlorine atom, located on + VII state of oxidation, however, the redox reaction rate involving perchlorate is very small and usually observed only in concentrated perchloric acid solution. This is probably due to the presence of oxygen atoms in a tetrahedral molecule that protects the reactive chlorine atom from the influence of reducers from environment [4]. For the same reason, perchlorate salts are poor complexing agents with metal ions, therefore they are widely used in analytical chemistry [14]. The hydration energy of perchlorate is among the lowest in popular inorganic anions which, in turn, has significant consequences in ClO4− determination methods, especially ion chromatography [37]. In acid solutions with concentrations of 0.1–4 M no reduction of perchlorate ions is observed by common reducing agents like thiosulphates, sulphides and iron (II) ions. As a result, perchlorate can accumulate in the environment for many years, undergoing very slow degradation [37].
Solid perchlorate are white or colorless crystals, hydrated (sodium perchlorate) or anhydrous (potassium perchlorate). Perchloric acid is a colorless, oily liquid. The solubility of perchlorate in water depends on the type of cation [1]. Most of them are well soluble in water and non-aqueous solvents, owing to their low affinity for metal cations [17]. Basic physical properties of the most commonly used perchlorates are shown in Table 2.
Ammonium perchlorate | Sodium perchlorate | Potassium perchlorate | Magnesium perchlorate | Perchloric acid 70% | ||
---|---|---|---|---|---|---|
Chemical formula | NH4ClO4 | NaClO4 | KClO4 | Mg(ClO4)2 | HClO4 | |
Molar mass (g/mol) | 117.49 | 122.44 | 138.55 | 223.23 | 100.46 | |
Physical state (25 °C) | White crystals with a rhombic structure | White crystals with a rhombic structure | Colorless crystal or white crystalline powder | White, hygroscopic powder | Colorless, odorless, oily liquid | |
Density (g/cm3) | 1.95 | 2.49 | 2.53 | 2.20 | 1.66 | |
Solubility g/100 g solv., 25 °C | Water | 24.92 | 209.60 | 2.06 | 99.60 | Miscible |
Methanol | 6.86 | 51.36 | 0.11 | 51.84 | ND* | |
Acetone | 2.26 | 51,75 | 0.16 | 42.89 | ND* | |
Melting point (°C) | 150, decompose | 468 | 400 | 251, decompose | −112 | |
Boiling point (°C) | – | 482 (monohydrate) | 600 | – |
* - No Data (the authors found no information of selected parameters)
Formation of perchlorate in drinking water systems
Perchlorate prevalence in drinking water may be caused by chlorine or gaseous ClO2 which are commonly used to disinfect water. The thermodynamically favorable reaction of perchlorate formation is given below [37]:
However, the reaction still lacks scientific evidence. The mechanism of reaction is probably more complex and requires intermediate steps [37]. An evidence of ClO4− formation during electrochemical oxidation of water was described [41]. Perchlorate would be generated in household bleaches, which contains up to 15% of sodium hypochlorite, NaOCl by disproportion in two-step reaction [37]:
Although the most likely potential pathways of perchlorate formation in drinking water have been proposed, little is known about contribution of ClO4− from the aforementioned sources [42].
In particular the reactions requires alkaline pH and they do not occur in ambient temperature very fast [37]. On the other hand, the electrochemical water treatment may lead to perchlorate contamination as by-product. There is a report [43] of concentrations up to 9 mg/L ClO4− obtained in disinfected water, much higher than drinking water equivalent level of reference dose (24.5 μg/L) [44].
Methods of production
Perchloric acid was first obtained in 1816 by von Stadion as a result of the low pressure distillation of mixture of concentrated sulfuric acid and potassium chlorate. In 1818 the same author described the method of obtaining HClO4 as a result of saturated aqueous chlorine dioxide solution [13], [14]. In both cases a solution of approximately 70% was obtained due to azeothropic mixture forming [14]. Current industrial production methods are based on the chlorates electrolysis to perchlorate. The basic substrates for the production are gaseous chlorine and caustic soda (NaOH) (1). Then hypochlorous acid (HClO) is formed and sodium hydroxide solution used in excess reacts with the obtained acid to give its sodium salt (2). Sodium hypochlorite then undergoes an electrolysis process to form NaClO3 (3) which, due to further electrolytic oxidation, gives NH4ClO4 (4). At last, followed by reaction with sulfuric acid or hydrochloric acid, HClO4 is formed (5) [45], [46]. The above mentioned reactions (1)–(5) are provided below.
Methods of determination
There are numerous techniques for determining perchlorate, although most of them are currently insufficient due to their relatively high limit of detection (LOD) and limit of quantification (LOQ). They include gravimetric, colorimetric, volumetric, spectrophotometric, potentiometric, capillary electrophoresis, yet ion chromatography methods seems to be most popular now, especially coupled with mass spectrometry detection. Due to good perchlorate solubility gravimetric methods appear to be problematic, yet there is some scientific data on this technique. Perchlorate can be determined in the complex with nitrone or tetraphenylphosphonium perchlorate [47], [48] or after reduction to chloride ions in form of silver chloride [49]. Detection limit of these methods is about 10 mg/L, so the sensitivity is too low for current applications and needs. Determination of perchlorate as a complex form with some colorants such as methylene blue, neutral red etc. is also used in colorimetric [50] and spectrophotometric [51], [52], [53], [54], [55] methods. However, there are many interfering factors which encumber the application of such methods in biological samples (e.g., body fluids). The range of detection limit of these methods is from 20 to 2 mg/L. There are some reports of volumetric determination of perchlorate, with titration of exceed perchlorate reducing agent like Fe2+ or Ti3+ [56], [57], [58]. There is a report [59] of a sensitive stripping voltammetric method based on voltammetric ion - selective electrode, with detection limit of 0.02 μg/L. A comprehensive review of perchlorate determination methods has been published 10 years ago [60]. Most of the current applications of perchlorate determination in various environmental and clinical samples are based on ion chromatography. The first modern perchlorate determination using ion chromatography (IC) were described in 1997 [61], [62]. IC has many advantages including low detection limits and determination at μg/L and requires only small sample volumes. There are many methods of sample preparation adapted for IC and the possibility of using different detectors. Application of tandem mass spectrometer as a detector results in improving selectivity and sensitivity of the ion chromatography by 100–1,000 times, with the reported detection limit of 0.5 ng/L [63]. IC and IC-MS/MS methods are extensively described elsewhere [64]. For the purposes of this review only the US Environmental Protection Agency (US EPA) methods are presented (see Table 3).
Standardized perchlorate determination Methods (US EPA).
Method | Limit of detection | Method number (EPA) | Year of description | References |
---|---|---|---|---|
IC with background conductivity suppression | 4 mg/L | 314.0 | 1999 | [65] |
IC with background conductivity suppression and pre-column compaction | 0.140 mg/L | 314.1 | 2006 | [66] |
Two-dimensional IC with suppression of background conductivity | 0.055 mg/L | 314.2 | 2007 | [63] |
LC – ESI – MS | 0.022 μg/L | 331.0 | 2005 | [67] |
LC – ESI – MS | 0.100 μg/L | 332.0 | 2005 | [68] |
Abbreviations: IC – ion chromatography, LC-ESI-MS – liquid chromatography – electrospray ionization – mass spectrometry.
Methods of remediation of perchlorate from the environment
Since the discovery of high concentrations of ClO4− in the waters of the Colorado River, USA in the late 1990s [1], many studies were conducted to find a close relationship between the presence of these compounds in the environment with human activity (industrial production, import of Chilean nitrate). In recent years, a large amount of work focuses on the natural occurrence of perchlorate in the environment. In connection with the appearance of new reports [69], [70], [71], [72], [73] on the occurrence of perchlorate in water samples, soils, vegetables, dairy products and other food products, there is a need to degrade the ClO4− anion to other non-toxic substances or to physically remove it from the environment. Due to high mobility of ClO4− in aqueous solutions, as well as its low reactivity, caused by high energy of activation and weak complexing properties, it is necessary to develop methods for decontamination of perchlorate on a large scale, because the use of conventional techniques such as coagulation, sedimentation and precipitation is expensive and inefficient [37]. In order to reduce or eliminate the threat caused by the presence of perchlorate in the environment, modern remediation methods are developed and improved.
Physicochemical methods include filtration, membrane processes such as electrodialysis, reverse osmosis, ultrafiltration and nanofiltration, adsorption on granular activated carbon, chemical and electrochemical reduction and, above all, ion exchange. Note, that only the last technique is used on a larger scale [74], in particular, for the removal of perchlorate from drinking water. The resins used to exchange perchlorate are essentially composed of a positively charged polymer (e.g., quaternary ammonium compounds) and related anions (e.g., chloride ions), which during the washing of the resin go into solution and ClO4− ions are retained on the resin surface. It has been shown that for the efficiency of the entire ion exchange process, the balance between kinetics and process selectivity is of great importance. Two-functional resins were developed in which two different quaternary ammonium compounds were used, differing in the chain length of the substituents. The longer chain caused selectivity, while the shorter one was responsible for improving the reaction kinetics. A method of regeneration of such resins was also developed, using FeCl4− ions obtaining almost 100% recovery, additionally consuming very small amounts of water [37], [75]. Other techniques are complicated to use, require expensive equipment and cause a large amount of waste that is difficult to dispose of [75].
For remediation of perchlorate by biological methods, selected species and microbial strains are used, which in the presence of a suitable electron acceptor (e.g., ClO4−, NO3−, O2) and carbon sources, can enzymatically convert perchlorate to other, non-toxic compounds, thus reducing the level of harmful substances to a level acceptable for drinking water. In general, perchlorate reducing bacteria can be divided into four main groups: (1) perchlorate reducting bacteria (PRB), which can reduce both chlorates and perchlorate. They belong to the genus Dechloromonas and Azospira. It is a group currently of the greatest importance in the processes of biological remediation of perchlorate. (2) bacteria that reduce chlorate (chlorate reducting bacteria, CRB), having enzymes that only reduce chlorates. Representatives are Ideonella dechloratans, Pseudomonas chloritidis-mutans ASK-1.3 a group of bacteria accumulating chlorate (high chlorate accumulating perchlorate reducing bacteria, HCAP), which reduce both chlorates and perchlorate, additionally have the ability to accumulate chlorates, which can be used by the symbiotic bacteria PRB and CRB. This group includes bacteria from Dechlorosoma species HCAP-C. (4) the denitrifying bacteria belonging to the family of Rhodobacter species Haloferax denitificans and Paracoccus halodenitrificans. For these bacteria the main source of energy are nitrogen compounds, in conditions of their lack they can use perchlorate as a substrate for the reaction instead, but their importance in remediation perchlorate is low [74].
Perchlorate – the origin and role of the human body
Routes of exposure
Toxins can enter the body in various ways. For perchlorate, there are potentially three possible routes of exposure: transdermal, inhalation and oral.
The transdermal route is the least important. As it is known perchlorate (in the most common chemical forms) in aqueous solutions are practically fully dissociated and ionized substances are very poorly absorbed through the skin due to epidermal barrier that effectively blocks the absorption of ions into the skin and further into the bloodstream. Therefore, it seems obvious that the potential of perchlorate entering the body through the use of contaminated water for hygiene purposes is small [37].
The inhalation route involves inhaling dust particles along with the air on which perchlorate molecules are adsorbed. In particular, perchlorate factory workers and the population of large cities where smog phenomenon occurs are the most vulnerable groups to this type of exposure [6]. Several studies [76], [77] conducted on the factory workers, who inhaled dust containing subclinical doses of perchlorate for a few hours a day showed no significant changes in the level of thyroid hormones in the blood compared to the control group, despite the increase in the content of perchlorate excreted in the urine. Study from China also informs about the increased amount of ClO4− in the urine of people exposed to inhalation contaminated with dust perchlorate. It was estimated that in the groups of toddlers, children and adults the contribution of perchlorate from indoor dust is 26, 28 and 7% of the estimated total daily intake, respectively, which makes it a significant route of exposure, especially in developmental age [78].
The oral route of exposure is the best known to date and seems to be the most important from all methods of absorbing perchlorate from the environment. Perchlorate is quickly picked up from the stomach and small intestine and then enter the bloodstream. Because of its good solubility and mobility in the aquatic environment, it can be found in many foods consumed by humans. The problem of contamination of drinking water with perchlorate was described in 1997 [1], while in recent years there has been an increasing interest in perchlorate in food products such as vegetables, fruits and even dietary supplements [9], [79]. Due to the fact that adverse effects of chronic admission of small doses of ClO4– for the functioning of the body are still unknown, in 2005, the US National Academy of Sciences (NAS) established a daily dose of perchlorate, which does not cause observable adverse effects at the level of 0.0007 mg/kg body weight [44], [80] and acceptable concentration of ClO4− ions in drinking waters at the level of 24.5 μg/L [81].
Absorption, distribution, metabolism, excretion (ADME)
In aqueous solution perchlorate is found in ionic form and transmitted into the blood stream from the gastrointestinal tract. Perchlorate is a poor complexing agent, therefore it is poorly accumulated in the human body. In the blood it is transported bonded with albumin, its half-life in human serum is about 6–8 h [3]. The only organs in which the perchlorate concentration to tissue concentration ratio is >1 are the thyroid (5–10x) and the skin (1–2x) [8]. When taken orally, it is absorbed very quickly and after about 10–15 min its presence in the urine can be detected. Elimination of perchlorate from the thyroid gland is relatively fast, in animal studies the half-life of ClO4− in the thyroid is about 10 h. Perchlorate is not metabolized in the human body and is also not a metabolic by-product. Some studies did not provide evidence for energetic alterations associated with perchlorate exposure at concentrations that are higher than those typically found in groundwater or surface water in the environment [82]. Thirty percent of the oral dose of perchlorate is excreted in the urine within 3 h of administration, 65–70% after 24 h, in over 95% after 48 h, hence their presence in urine is an important biomarker of exposure to ClO4− [7]. The remaining portion is removed from the body in other ways, e.g., through sweat glands and with breast milk [83].
Perchlorate in various matrices
General sources of perchlorate in environment were described in 1.1. As it is mentioned above, the most common route of exposure is via digestion system. The main source of perchlorate in human body involves contaminated water and food [16]. Numerous studies concern of perchlorate levels in ground, surface and drinking water. In the US nearly 4,000 of public water systems were surveyed and found that in 4.1% of them perchlorate concentration was above 4 μg/L. [84].
A review published in 2018 [85] has been focused on global perchlorate pollution of water. The authors concluded that most of the peer-reviewed studies (n=39; 51%) were conducted in USA and Canada and further studies in different geographical regions seem to be essential [85]. An example from India, where mean concentration of ClO4− in bottled water was 93.19 μg/L can be a confirmation of worldwide perchlorate contamination studies needs [86]. An analysis of isotopic composition of perchlorate is useful to distinguish naturally formed from man-made ClO4− [87]. Mobility of perchlorate in soils depends on many factors, such as its concentration, chemical composition and aridity of the ground etc. [88]. Although arid or semi-arid soils were investigated [8], still little is known of the presence of ClO4− in agricultural soils. Study by Calderon et al. [88] found that fertilizers, especially nitrogenous could be contaminated with perchlorate (range 12.8–95.3 mg/kg). In this way perchlorate may be absorbed to soil [88]. It is known that various plants can absorb perchlorate from soil and accumulate it in their tissues in higher concentrations than found in ground [87]. There are some studies concerning the risk of exposure to ClO4− via ingestion of fruits and vegetables. In general, the highest level of perchlorate was found in apricot from Chile (145.650 ± 4.031 μg/kg), cantaloupe form Guatemala (463.500 ± 6.364 μg/kg), spinach from USA, Mexico and Korea (39.9–187 μg/kg) [69], [89], [90]. Processed food from China was also investigated and it was found mean 6.1 μg/kg. Calculated estimated daily perchlorate ingestion for adults (EDI) of 488.5, 523.8 and 501.2 ng/kg/ bw/day in three different regions did not exceed the reference dose 700 ng/bw/day [91], but the tolerable daily intake (TDI) proposed by European Food Safety Authority (EFSA) of 300 ng/bw/day [92] was overrun. What is more, the collected data suggest that contribution of perchlorate from dietary products in relation to drinking water is near 4:1 and this ratio may be higher in children [93].
According to the scientific opinion on the risks to public health related to the presence of perchlorate in food, in particular fruits and vegetables (EFSA, CONTAM panel, 2014) [92] it is recommended to monitor the presence of perchlorate in food. The CONTAM Panel concluded that more data is needed on the occurrence of perchlorate in food in Europe, in particular for vegetables, infant formula, milk and milk products, to further reduce uncertainty in risk assessment. High levels of perchlorate have been found in the Cucurbitaceae and leafy vegetables, especially those grown under glass or sheltered [92].
Another 2017 CONTAM report confirmed that exposure to perchlorate may pose a risk to public health, so the European Commission has finally established maximum levels for perchlorate for foods containing significant amounts of perchlorate and for foodstuffs relevant to the possible risk of exposure to perchlorate. Exposure of particularly vulnerable population groups such as infants and young children [94].
On May 25, Regulation (EU) 2020/685 was published, amending Regulation (EC) No 1881/2006 concerning maximum levels for perchlorate in certain foodstuffs. In the European Union, Regulation 2020/685 applies from 1 July 2020. According to it, maximum levels for perchlorate are established for the following foodstuffs: fruit and vegetables: 0.05 mg/kg, except Cucurbitaceae and kale: 0.10 mg/kg; leafy vegetables and herbs: 0.50 mg/kg; tea (Camellia sinensis), dried: 0.75 mg/kg; herbal and fruit infusions, dried: 0.75 mg/kg; infant formulas, follow-on formulas, food for special medical purposes intended for infants and young children and infant formulas: 0.01 mg/kg; baby food: 0.02 mg/kg (young-child formulas are milk-based drinks and similar protein products intended for young children. These products are outside the scope of Regulation (EU) No 609/2013 (Report from the Commission to the European Parliament and the Council on infant formulas [COM/2016/0169 final]), and processed cereal products: 0.01 mg/kg (foodstuffs listed in this category as defined in Regulation (EU) No 609/2013 of the European Parliament and of the Council of 12 June 2013 on foods intended for infants and young children) [95]
Effects on the thyroid gland and secretion of thyroid hormones
Thyroid is the critical organ for exposure to perchlorate. The effect of perchlorate on the thyroid gland was discovered in the 1950s and 1960s, during which period these compounds were used in the treatment of hyperthyroidism [3]. Perchlorate belong to a group of compounds called goitrogens. Apart from perchlorate, goitrogens include thiocyanate (SCN−) and nitrate (NO3−) and some other organic compounds. These compounds have the ability to inhibit the iodine uptake by the thyroid cells [96].
Iodine is necessary for the synthesis of thyroid hormones: 3,5,5′-triiodothyronine (T3) and 3,5,3′,5′-tetraiodotyronine (thyroxine, T4). Iodine is transported actively from the bloodstream to the follicular cells in the form of iodide ions due to the mediation of the membrane protein sodium-iodine symporter (NIS). The transport of iodides to the thyroid meets the electrochemical and metabolic criteria of active transport. It is an oxygen-dependent process which does not occur at low temperature and is also dependent on adenosine triphosphate (ATP). The first reports on the dependence of iodine transport to the thyroid in the presence of Na+ ions appeared in the 1950s [3]. Currently, a model in which the NIS enzyme transports two Na+ ions and one I− ion into the cell is proposed. The presence of NIS in the tissue of the thyroid gland enables the flow of iodides from the environment, in which their concentration is very small (10−8–10−7 M), to thyroid cells, where the prevailing concentration is several hundred times greater. Such high levels of iodide are probably necessary for proper synthesis of hormones, T3 and T4, since patients who have been recognized to impair the ability to concentrate iodine suffer from the goiter and hypothyroidism. An important and characteristic feature of the transport of iodides to the thyroid gland is the high specificity of the enzyme for iodide ions in reference to the much higher concentrations of chloride ions. Studies have shown that the iodine ion is not the most specific anion for NIS, and a series of affinities are as follows: TcO4− ≥ ClO4− > ReO4− > SCN− > BF4− > I− > NO3− > Br− > Cl−. It is noteworthy that the presented order of ions is also a lyotropic series or a series of Hofmeister [4], [97]. However, it is not entirely clear which anion property determines this series (crystal radius, hydration enthalpy, etc.). Some anions (e.g., I−, SCN−, NO3−) are easily transported via NIS [98]. In case of perchlorate there is some empirical data that ClO4− may be translocated by symporter, especially in milk glands. Perchlorate was described as NIS most potent blocker, without possibility of NIS mediated transport [99]. However, latest studies shown that perchlorate inhibit iodide transport in more complex way than by the competition only. Low concentrations of ClO4− inhibit I− transport also by changing the stoichiometry of I− transport to 1:1, which diminishes the driving force. Electrophysiological experiments suggested that ClO4− ions may bind to a high-affinity non-transport allosteric site in NIS that prevents Na+ from binding to one of its two sites [11]. The harmful effect of perchlorate associated with the inhibition of iodine transport to the thyroid gland is closely related to the disturbed production of hormones T3 and T4 by this gland. The synthesis of these hormones is a complex process, depending on many factors. Inhibition of the transport of iodide ions to the lumen of the thyroid vesicle blocks the key stage of the synthesis of T3 and T4, which is the iodination of the tyrosine and its iodinated derivatives to the proper hormones. The general function of T3 and T4 is based on stimulation of oxygen consumption in the body cells, causing the increase of protein synthesis and obtaining a positive nitrogen balance. The result of their action is a general increase in the metabolic rate of carbohydrates and fats. They are also important modulators of developmental processes, by stimulating the pituitary gland to secrete growth hormone (somatotropin), as well as influencing the development of lungs, bones, blood vessels and CNS, which is why the group that is particularly exposed to iodine and hypothyroidism are women during pregnancy and postpartum as well as children [83], [96], [97].
Hypothyroidism manifested in fetal life or in infancy can lead to cretinism, which is characterized by low birth weight, numerous irreversible developmental defects, both physical and mental. In adults, the most common symptoms include heart failure, drowsiness, constipation, hypersensitivity to cold, brittleness of the hair and general weakness of the body [83]. Several independent recent studies suggested that various presented symptoms pertinent to autism might correlate with iodine status [100], [101].
Other toxic effects of perchlorate exposure
NIS is found not only in the tissue of the thyroid gland, its presence was also detected in eyelid body, cerebrovascular plexus, salivary and lactic glands, the latter especially during lactation. In very small amounts, NIS can be found in cellular membranes in the tissue of the heart, kidneys and lungs [99]. Unlike the thyroid gland, iodine is not metabolized in these tissues and it is reabsorbed into the circulatory system or in case of secretory glands to saliva and milk [102]. With the exception of the mammary gland, the function of iodine in these structures is poorly known and it seems that the disturbance of its transport by perchlorate does not cause changes in their physiological functioning. Doses of perchlorate used in the treatment of hyperthyroidism (400–2,000 mg/day) were fairly well tolerated by the majority of patients, there are some reports of gastrointestinal disorders, skin rashes, enlargement of the lymph nodes and fever. Studies concerned about 3–4% of patients taking small doses (400–600 mg/day), while in patients receiving higher doses (1,000–2,000 mg/day) this percentage increased to 16–18%. From severe side effects, sporadic aplastic anemia or agranulocytosis (13 people) have been reported, seven people died, and the total number of patients treated with hyperthyroidism with potassium perchlorate was greater than 1,000 [103], [104], [105], [106], [107].
Research methods
For the purpose of this review the epidemiological data were collected from January 2010 to July 2020. The review involves exclusively items selected from peer-reviewed publications written in English language. Importantly, the choice of the period covered by the review was dictated by the willingness to present the most current data and to show various directions towards which researches are currently conducted. Data collection was conducted using guidelines that comprise with Preferred Reporting Items for Systematic Reviews and Meta-Analyses (PRISMA) and a flow chart of article selection process was adopted [108].
As the literature search engine, the browsers in the Scopus, PubMed and Web of Science databases were used. In addition, the results displayed as “relevant” were also investigated into.
The following inquires have been used: “perchlorate AND human health” and this phrase in conjunction with “cancer” and “disease”, “perchlorate AND toxicity”, “perchlorate AND thyroid” and this phrase in conjunction with “hormones” and “hypothyroidism”, “perchlorate AND neurodevelopment”, “perchlorate AND environment”and this phrase in conjunction with “contamination”, “perchlorate AND determination” and this phrase in conjunction with “body fluids”, “urine”, “blood”, “serum”, “plasma”, “food”, “water”, “perchlorate AND goitrogens”. In order to qualify the work for the review, the following exclusion criteria were applied:
works that do not contain original data, e.g., reviews, comments
works which have not been independently peer-reviewed e.g., conference speeches, posters, technical reports, letters, etc.
animal studies
molecular research on genes, tissues and cell cultures
individual medical cases
The collected data included names of authors, journal, year of publication, country, type of study, sample size, type of health impact, statistical analysis and other critical remarks.
Eight hundred and seventy-five unique records were found as a result of the search operations, out of which 772 items were excluded on the basis of the exclusion criteria. Finally, 103 works were qualified for the critical review of the entire text and 29 of them were included in this review (Figure 1).
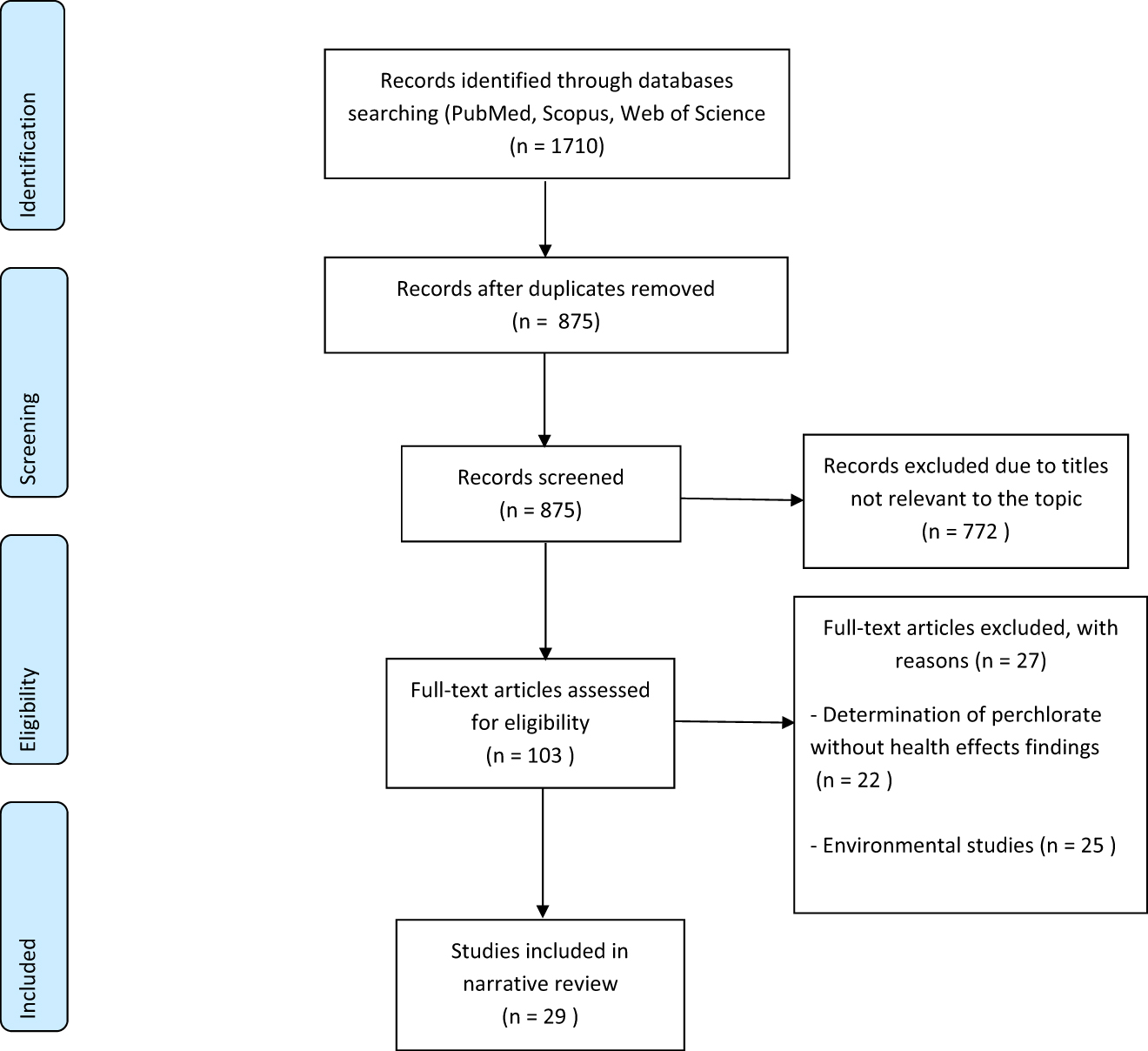
Flow chart of article selection.
Results and discussion
Over the years 2010–2020, many papers have been published relating to various aspects of perchlorate exposure. An important issue was to properly assess the impact of perchlorate on the level of thyroid hormones in pregnant women owing to the fact that already in 2005, the US National Research Council (NRC) suggested that they, just like newborns and infants, are the most sensitive to the harmful effects of goitrogens [44]. In 11 works from this period urine perchlorate concentrations and levels of thyroid hormones and TSH in the blood were determined. Most studies also included iodine concentration in urine.
Thyroid function studies
No significant associations found
Pearce et al. over the years 2010–2012 published three papers [109], [110], [111] in which correlations between perchlorate and thiocyanate content and iodine concentration in urine were studied as well as the levels of thyroid hormones in pregnant women in various parts of the world. The authors measured these parameters in pregnant women in the first and second trimester of pregnancy living in Turin, Italy and Cardiff, United Kingdom. An additional criterion was the division on grounds of thyroid gland diseases - both groups were divided into normal thyroid function group and those who suffered from hypothyroidism. The median (range) concentrations were described: 98 (12–847) μg/L iodine and 2.1 (0.03–368) μg/L perchlorate in urine in hypothyroidism group and 117 (2–497) μg/L iodine and 2.6 (0.3–49) μg/perchlorate in the urine in control group in Cardiff. The median (range) concentrations in Turin were as follows: 55 (12–1129) μg/L iodine and 5.04 (0.04–108) μg/L perchlorate in urine in hypothyroidism group and 50 (6–584) μg/L iodine and 5.2 (0.2–168) μg/L perchlorate in urine in control group. Even though there was no significant correlation between perchlorate concentration in urine and thyroid hormone levels in the blood plasma, the study showed significant iodine deficiency in the studied population in Italy [109]. In next work the authors compared levels of goitrogens including perchlorate, iodine and thyroid hormones in pregnant women in the first trimester of pregnancy originating from Los Angeles, USA and Cordoba, Argentina. Perchlorate levels in urine were median (range) 7.8 (0.4–284) μg/L and 13.5 (1–676) μg/L in Los Angeles and Cordoba, respectively, and iodine levels were found 144 (16–733) μg/L and 130 (9–693) μg/L in Los Angeles and Cordoba, respectively. No statistically significant relationship was found between the concentration of perchlorate in the urine and the level of thyroid hormones in the blood, however, attention was paid to a significantly higher (p<0.003) perchlorate level in women in Argentina [110]. The third study was carried out in Athens, Greece, where women in the first trimester of pregnancy were examined analogously to the previous ones in order to determine the concentrations of perchlorate, iodine and thyroid hormones. It was found 4.1 (0.2–118.5) μg/L median (range) of perchlorate and 120 (28–538) μg/L of iodine in the urine, respectively. With respect to the entire studied population, a significant relationship was found between free T3 (FT3) and free T4 (FT4) levels and urinary perchlorate concentration, yet in the subgroup of women with iodine concentration <100 μg/L in the urine this relationship was not demonstrated [111]. All perchlorate determinations were performed using the IC-ESI-MS technique described elsewhere [112]. The measurement sensitivity was sufficient, the detection limit was 0.003 μg/L, and the measurement range of concentration was 0.025–100 μg/L. The authors of the above studies concluded that perchlorate have no significant effect on thyroid functions when exposed to environmental conditions. Importantly, there is a relatively small number of participants in each study. Note that the largest number has been studied in Pearce et al. [109], the smallest in Pearce et al. [111] (see Table 4). Additionally, there is a lack of estimated daily dose of perchlorate taken by the examined patients. No statistically significant relationship between perchlorate content in urine and thyroid hormone levels in pregnant women was also found in the paper by Leung et al. [113], where the concentration of perchlorate in breast milk was studied as well. The following perchlorate concentration results were obtained in milk, urine of mothers and urine of infants: 4.4 (0.5–29.5), 3.1 (0.2–22.4) and 4.7 (0.3–25.3) μg/L median (range), respectively. Concentrations of iodine in milk, in urine of mothers and in urine of infants was as follows 45.6 (4.3–1,080), 101 (27–570) and 197.5 (40–785) μg/L median (range), respectively. However, these studies were based on a population from one selected area (Boston, USA), a small sample size (N=64 mother-child pairs) and a socioeconomic group declaring an appropriate diet, especially in the context of sufficient iodine intake. It seems, therefore, that the results obtained in this study cannot be unambiguously extrapolated to estimate the effect of perchlorate on the level of thyroid hormones in breastfed babies. Mortensen et al. [114] investigated the effect of perchlorate, thiocyanate and nitrate on the plasma thyroid hormone concentration in women in the third trimester of pregnancy. The study group consisted of 359 women from various locations in the United States. The results of concentrations 4.03 (3.72–4.36) μg/L geometric mean, (95% CI) of perchlorate and median (range) 167 (107–217) μg/L of iodine in urine were obtained, with no significant relationship between perchlorate concentration in urine and the level of TSH and FT4; however, iodine deficiency in the study population has not been found as well. Gold et al. [115] examined the impact of environmental exposure to perchlorate in the population with various levels of exposure, and there was no correlation between the level of exposure and thyroid function, however, data on the concentration of perchlorate and iodine in urine were not presented by the authors.
Correlation studies between perchlorate exposure and plasma thyroid hormone levels.
Lp. | Objective | Number of participants | Method of ClO4−determination | Level of ClO4− (median (range) µg/L) | Level of iodine (median (range) µg/L) | ClO4− and thyroid hormone associations | References |
---|---|---|---|---|---|---|---|
1 | Assessment of environmental perchlorate impact on thyroid function during pregnancy among cohort from cardiff, UK and turin, Italy | 374-Hypothyroid | IC-ESI-MS | 2.1 (0.03–368)-hypothyroid | 98 (12–847)-hypothyroid | No significant associations | [109] |
480–euthyroid (UK) | 2.6 (0.3–49) – euthyroid (UK) | 117 (2–497) – euthyroid (N=383) (UK) | |||||
261-Hypothyroid | 5.04 (0.04–108)-hypothyroid | 55 (12–1129)-hypothyroid | |||||
526-Euthyroid (Italy) | 5.2 (0.2–168) – euthyroid (Italy) | 50 (6–584) – euthyroid (Italy) | |||||
2 | To assess the impact of maternal perchlorate exposure via drinking water on newborn thyroid function | 451,708 – newborns unexposed group (<5 µg/L perchlorate in drinking water) | NA | NA | ND | Statistically significant higher level of TSH in newborns from exposed group compared with unexposed subjects | [131] |
45,750 newborns exposed group (>5 µg/L perchlorate in drinking water) | |||||||
3 | To assess the risk of exposure on perchlorate in children | 92 children aged 1–12 months | IC-MS | 1.21 (0.86–1.69) GM (95% CI) | 130.3 (112.8–150.5) GM (95% CI) | Significant positive association with TSH in children with low iodide, positive correlation with T4 in whole population | [123] |
4 | Association between levels of urinary iodine and perchlorate and TH status in pregnants from different countries | 134–Los Angeles | IC-ESI-MS | 7.8 (0.4-284) – LA | 144 (16-733) – LA | No correlation with TSH, T3 and FT4 Index | [110] |
107–Cordoba | 13.5 (1-676)-cordoba | 130 (9-693)-cordoba | |||||
5 | Association between levels of perchlorate and biomarkers of its exposure and thyroid hormones | 970 – men | IC-MS/MS | 4.3(1.0–18.4) (GM 5-95% CI) – men | 174(43–742) (GM 5-95% CI) – men | Significant negative association with T3 and T4 | [117] |
907-Women | 3.4(0.7–15.6) (GM 5-95% CI)-women | 145(31–567) (GM 5-95% CI)-women | |||||
6 | Assessment of relationships between environmental perchlorate and thiocyanate exposure and infant serum thyroid hormone levels | 64 women and their 1-3 month-old breastfed children | IC-MS | 4.4 (0.5–29.5) – mothers, breast milk | 45.6 (4.3–1080) – mothers, breast milk | No significant correlation between TSH and FT4 | [113] |
3.1 (0.2–22.4) – mothers, urine | 101 (27–570) – mothers, urine | ||||||
4.7 (0.3–25.3) – infants, urine | 197.5 (40–785) – infants, urine | ||||||
7 | To assess the adverse effects of perchlorate exposure on pregnant woman from Athens, Greece | 134 pregnant woman | IC-ESI-MS | 4.1 (0.2–118.5) | 120 (28–538) | No significant association with serum TSH, weak significant negative association with serum FT3 and FT4 | [111] |
8 | To assess the impact of occupationally perchlorate exposure in ammonium perchlorate factory workers in China | 51 – exposed group | UHPLC-MS/MS | 8.89 (0.92–24.71) – exposed group | 155.4 – exposed group | No significant associations | [129] |
41 – control (unexposed) group | 5.56 (0.37–20.55) – control group | 161.0 – control group | |||||
9 | Impact of perchlorate and other goitrogens exposure on thyroid hormone levels among different risk groups (A, B, C) | 390 – group A (low exposure) | IC-MS/MS | A – 1.8(0.36 – 2,74) | A – 163(100-762010) | Significant negative association with T4 | [118] |
553 – group B (moderate exposure) | B – 4.02(2.75 –5.8) | B – 206(101–50502) | |||||
64 – group C (high exposure) | C – 9.15(5.82–47.80) | C – 80(37–99) | |||||
10 | To assess potential risk of thyroid disruption caused by environmental high perchlorate level | 60 – area A (exposed) | IC-MS | ND | ND | No significant association | [115] |
58 – area B (potentially exposed) | |||||||
63 – area C (unexposed) | |||||||
11 | Impact of serum levels of perchlorate and thiocyanate on serum levels of serum TH among high and low exposure groups | 83 – e-waste recycling workers | LC-MS/MS | 0.120 (<0.050 –1.25) – e-waste workers, serum | 3.25(1.26–12.2) – e-waste workers, serum | No significant associations | [130] |
48 – control group living in rural site | 0.086 (<0.05 –0.660) – rural site residents, serum | 2.89(1.32–13.1) – rural site residents, serum | |||||
12 | To assess impact of environmental perchlorate and thiocyanate exposure on thyroid function in pregnant women | 200 first trimester pregnant women | IC-MS | 1.9 (0.1–35.5) | 153.5 (50–1219) | Significant positive correlation with TSH and negative with FT4 after adjustment on perchlorate/creatinine ratio | [120] |
13 | To assess the impact of combined effects of major NIS inhibitors on FT4 index in subjects with low <100 ug/L urinary iodine status | 1875 (890 – men, 985 – women) – NHANES 2001–2002 | IC-MS/MS | NA | NA | Significant negative association between urinary perchlorate and serum FT4 among non-pregnant woman. | [147] |
2568 – non-pregnant women and 49 – pregnant – NHANES 2007–2008 | |||||||
14 | Correlation between co-exposure to perchlorate, nitrate and thiocyanate and thyroid function | 284 pregnant women | IC-MS/MS | 3.54 + 0.02 (mean + SE) µg/g creatinine | 235.39 + 39.40 (mean + SE) µg/g creatinine | Significant positive association with TSH after creatinine adjustment | [121] |
15 | To assess impact of perchlorate exposure via drinking groundwater on thyroid hormone levels in cord blood in infants from Iran | 25 neonates | NA | NA | NA | No significant correlation between cord blood TSH, T3, T4 and perchlorate concentration in drinking water | [132] |
16 | To evaluate possible adverse effect of low level perchlorate exposure on thyroid function | 1880 first trimester pregnant women | IC-MS/MS | 6.50 (0.23– 177) | 154.5 (0.8–3000)–1818 subjects studied | Significant positive correlation between log perchlorate concentration and log TSH serum concentration, significant negative correlation between log perchlorate concentration and T4 and F T4 serum concentration | [122] |
17 | To examine the impact of major NIS inhibitors on serum thyroid indicators | 359 | IC-MS/MS | 4.03 (3.72-4.36) GM (95% CI) | 167 (107–217) | No significant associations | [114] |
18 | Association between maternal environmental perchlorate exposure and birthweight and preterm birth in their offspring | 1957 mother – newborn pairs | IC-MS/MS | 8.57 + 9.99 (mean + SD)-mothers | 216.61 + 256.81 (mean + SD)-mothers | Significant negative correlation between T4 FT4 and positive correlation between TSH in 862 male births (subjects which provide sufficient data) | [125] |
19 | Impact of perchlorate and thiocyanate on thyroid function in pregnant women | 308 pregnant women | IC-MS | 2.1(0.3–143) | 88 (55–157) | Significant negative association with T4 | [124] |
20 | Perchlorate and other major NIS inhibitors influence on maternal and infant thyroid function | 185 mother – newborn pairs | IC-MS | 4.00 – mothers, urine, µg/g creatinine | ND | Significant positive correlation between | [116] |
2.30-mothers, colostrum | Colostrum perchlorate level and maternal TSH |
NA – not applicable, ND – no data, TH – thyroid hormone, TSH – thyroid stimulating hormone, T3 – triiodothyronine , T4 – thyroxin, FT3 – free triiodothyronine , FT4 – free thyroxin, SE – standard error, SD – standard deviation, GM – geometric mean, CI – confidence interval, LA – Los Angeles, CA – California, UK – United Kingdom, NIS – sodium/iodine symporter , NHANES – National Health and Nutrition Examination Survey, LC-MS – liquid chromatography – mass spectrometry, IC-MS – ion chromatography – mass spectrometry, IC-MS/MS – ion chromatography - tandem mass spectrometers, IC-ESI-MS – ion chromatography - electrospray ionization - mass spectrometry, LC-MS/MS – liquid chromatography - tandem mass spectrometry, UHPLC-MS/MS – ultra high performance liquid chromatography - tandem mass spectrometry.
Significant associations found
On the other hand, there are several papers that also described the effect of perchlorate concentrations in the body fluids on the level of thyroid hormones in the blood, indicating the existence of statistically significant relationships between these parameters. Most of the reviewed studies concerned to determine perchlorate in urine, although there is an interesting work that describes a positive relationship between the concentration of ClO4− in the colostrum and the level of TSH in mothers, nonetheless without indicating the significant correlation in the case of TSH in their children [116]. The results described in this paper cover only 185 mother-child pairs, so it seems to be insufficient to be representative of the general population.
Three reviewed studies were based on data collected during NHANES 2007–2008. Paper by Mendez and Eftim [117] uses specially designed additive models (GAMMs) to estimate the effect of perchlorate, phthalates and other NIS inhibitors on the level of thyroid hormones. In a sample of 970 men and 907 women, a significant negative correlation was found between the concentration of perchlorate in the urine and the concentration of triiodothyronine and thyroxine in the plasma. Another work [118] which is based on data obtained from NHANES 2007–2008 describes the effect of environmental exposure to perchlorate on thyroid hormone levels. The results indicate that people classified as high-risk exposures (1,939 people) had an average of 5% lower plasma concentrations of thyroxine than those from the low-risk group (2,084 people). Moreover, people with a high content of perchlorate, thiocyanate and low iodine concentration in the urine (n=62) had thyroxine at 12.9% lower (mean difference = 1.07 mg/dL, 95% CI=0.55–1.19) than in patients with low perchlorate, thiocyanate, and normal urine iodine (n=376). Undoubtedly, a great advantage of this study appears to be a numerous test group as well consideration of other factors i.e., thiocyanate and iodine, apart from perchlorate. Note that the research roughly confirms previous reports about the negative impact of NIS inhibitors on thyroid function.
Drawing on NHANES 2007–2008 Przybyla et al. [119] examined the relationship between the content of 11 different compounds (perchlorate, some phenolic compounds and phthalates) in the urine and the level of thyroid hormones. The study group consisted of 850 men and 710 women, with an mean concentrations 3.48 and 4.37 μg/L of perchlorate in the urine, respectively. What is interesting is that authors describe the negative impact on thyroid function (a significant negative relationship with the level of T4 in the plasma), when the cumulative effect of all tested compounds is taken into account. Additionally, it was observed only for men. The possible explanation given by the authors is the disruption of liver function, observed in animal studies, probably caused by mentioned goitrogens, which can reduce the conversion of T3 to T4. For the perchlorate itself, the statistically significant relationship was not confirmed.
Studies based on NHANES results have the advantage of a very large study group, but they provide results only for US population. There were a number of studies, where the authors examined some specific populations or they were focused on different than previously described objectives. Charatcharoenwitthaya et al. [120] determined these parameters in 200 women in the first trimester of pregnancy from Thailand, obtaining median (range) 1.9 (0.1–35.5) μg/L of perchlorate and 153.5 (50–1219) μg/L of iodine in the urine. There was a relevant positive correlation between TSH concentration and negative relationship between FT4 level in plasma and urine perchlorate content. What is more, previous studies by the authors [109], [110], [111] found no significant correlations. The differences between mentioned studies might be caused by higher risk of exposure on perchlorate in Thai group, especially via foodstuffs and drinking water. Horton et al. [121] have examined a 284 women in the first trimester of pregnancy in the area of New York, USA. The mean ± standard error concentrations of perchlorate and iodine in urine were 3.54 ± 0.02 mg/g creatinine and 235.39 ± 39.40 mg/g creatinine, respectively. A statistically significant relationship was found between the level of TSH in plasma and the concentration of perchlorate in urine calculated as creatinine. The undoubted advantage of this study is applying a novel statistical methodology (WQS) to assess combined effects of more than one thyroid disrupting factor. But relatively inconsiderable sample size and small study area can lead to the risk of bias. The studies on much larger samples than mentioned above were carried out by Steinmaus et al. in 2016 [122]. Perchlorate and iodine in the urine and thyroid hormone level in blood plasma were determined in 1880 women in the first trimester of pregnancy in the area of California, USA. The median (range) level of 6.50 (0.23–177) µg/L perchlorate and 154.5 (0.8–3,000) µg/L of iodine in urine were found. It is interesting to note that the ClO4− concentration in the urine was almost twice higher than in the general population of the US. A significant positive correlation was found between the logarithmic values of urine perchlorate concentration and TSH in the serum and the negative correlation between the logarithmic T4 and FT4 values in serum and urinary perchlorate. The obtained dependences are in line with previous data obtained during NHANES 2002–2003. An unexpectedly strong correlation between the concentration of perchlorate (as well as thiocyanate and nitrate) in the urine and the level of T4 on a sample of 92 children aged 1–12 months was described by Cao et al. [123]. In addition, perchlorate and TSH were positively related. Also, Knight et al. [124] confirms the critical negative correlation between urinary perchlorate concentration and serum FT4 level. What is more, there was no correlation between the level of ClO4− and iodine in urine. The study was conducted in the South West of England on a group of 308 women in the third trimester of pregnancy.
Association between perchlorate exposure and children gross measures
In a study of Knight et al. [124] in addition to the impact of perchlorate on the thyroid function, the birth weight of children was also measured and correlated with perchlorate level, but there was no significant correlation. The results are partly consistent with previous reports [125], where in a sample of 1,957 mother-child pairs, there are no significant relationships between perchlorate urine concentration and birth weight of children in the general population. However, a significant positive correlation was inspected in the case of newborns, especially in preterm births. An analogous relationship was not confirmed for girls. In addition, a significant negative relationship between the level of FT4 and T4 and positive for TSH in the plasma of newborns and the concentration of perchlorate in their mothers was described. Besides, the work of Evans et al. [126], where 107 mother-child pairs were examined. The study points to no relevant correlations between the concentration of NIS inhibitors (including perchlorate) in urine and the height and weight of newborns as well as the week of delivery. What is interesting, they report an impact of perchlorate and other major NIS inhibitors on the head circumference of newborns. The study found that every 2.62 ng of perchlorate per µg of creatinine in the urine results in a reduction of the head circumference by 0.32 cm, while in the case of the combined effect of perchlorate, thiocyanate and nitrate the head circumference increases by 0.48 cm for each 3.38 ng/g creatinine. These findings, although interesting, require further confirmation on a larger study group, especially as earlier reports by Blount et al. [127] point to the lack of dependence between the concentration of perchlorate in body fluids of mothers and their children, and the size, including head circumference and weight of newborns.
Interesting results are provided by the work of Mervish et al. [128], in which the influence of major NIS inhibitors on the weight and waist circumference of 940 girls aged 6–8 years from different areas of the USA (New York, Cincinnati, California) was examined. Although no direct correlation of perchlorate and these parameters was described, the increase in perchlorate, thiocyanate and urine nitrate concentrations was correlated with a slower waist and BMI increase. This contrasts with the results described above [124], [125], [126], [127], where newborns were studied, but no dependence was found, or they were found only in boys or only for head circumference.
Occupational risk assessment in factory workers
Apart from the research carried out in the general population, there are some studies concerned on occupational risk of perchlorate exposure in heavy industry employees. Chen et al. [129] investigated differences in perchlorate concentration in employees of the fireworks factory in China, who were exposed to perchlorate to varying degrees. The content of ClO4− in the urine of employees finishing their shift was median (range) 8.89 (0.92–24.71) μg/L for exposed group (51 people) and 5.56 (0.37–20.55) μg/L control group (41 people). There was no significant correlation between the level of thyroid hormone in the plasma and perchlorate concentration in the urine between the two groups studied, however the studied sample was very small (92 people in total). In addition, both groups showed sufficient iodine intake, its urinary concentration was 172 and 184 μg/L, respectively, for the control and studied groups, which most probably had an effect on normal thyroid function in the study population. Another study from the area of East Asia [130] where thyroid activity and perchlorate concentration in the blood plasma were compared in people working in the factory dealing with the processing of waste electronic equipment (e-waste) and the rural population, with a negligible degree exposure to perchlorate from the territory of Vietnam. Perchlorate concentrations in the plasma were median (range) 0.120 (<0.050–1.25) and 0.086 (<0.05–0.660) μg/L, respectively. Here too, no significant correlation has been examined between ClO4− concentrations and plasma thyroid hormone level, yet a significantly higher concentration of perchlorate in the exposed population has been recorded. These results and previously published data [7] suggest that the occupational risk of perchlorate exposure has minor impact on thyroid function. The main weaknesses of mentioned results are small sample size, only one geographical region (East Asia) studied and generally iodine-sufficient study groups. The choice of a non-standard perchlorate matrix (plasma) in some cases, where perchlorate are in very low concentration, make difficult to transfer the results to general population. It should be noticed that the one of the most vulnerable group to perchlorate exposure are pregnant women, but there is no evidence of occupational risk in such subgroup and there is high uncertainty of extrapolation results from healthy factory workers on pregnant women.
Health risk assessment of perchlorate concentration in drinking water
Two studies described the effect of environmental exposure to perchlorate, however, without directly testing the content of these compounds in biological materials (urine, plasma, other body fluids), but based on their concentration in drinking water. The study of Steinmaus et al. [131] was based on data from the area of California, where in 1998, 800 drinking water samples were tested to determine perchlorate concentration and 497,458 neonatal plasma samples to determine the level of TSH. Subsequently, plasma samples were assigned to water samples from the relevant site and classified as a high exposure group (45,750 people, ClO4− concentration in water >5 μg/L) and a low exposure group (451,708 people, the concentration of ClO4− in water <5 µg/L). In neonatal samples 24 h after delivery, the odds ratio (OR) for TSH level in plasma greater than 25 μIU/mL was 1.54, p<0.0001 in the high-exposure group, which confirms the existence of a positive correlation between TSH levels and exposure to perchlorate in newborns. A large sample is the undoubted advantage of this study, but the possible effect of other NIS inhibitors, which may also have a significant impact on the increase in TSH has not been considered. Javidi et al. [132] examined the impact of perchlorate exposure through drinking water in 25 newborn infants from the Iran area. No significant relationship was found between perchlorate concentration in water and thyroid function and TSH level in newborns, however, the study was conducted on a very small test group and thus requires the extension of a larger number of participants from this geographical region.
Other potential perchlorate exposure health risk
As it was mentioned iodine deficiency and disruptions in the thyroid hormones production may result in serious neurodevelopmental disorders in children. To our knowledge, the only research on the impact of exposure to perchlorate of pregnant women on the level of IQ in their children during the time period of interest of this article have been described by Taylor et al. [133]. In a sample of 487 mother-child pairs from two different European countries: Italy and Great Britain, it was proven that the concentration of perchlorate in urine of mothers with 10% of the highest results yields a significant increase in the chance of finding their child in the group of 10% of the lowest IQ results at the age of three. Moreover, the stronger relationship was described for verbal IQ than for non-verbal IQ. It should be added that the research concerned only mothers with diagnosed hypothyroidism, there are no such studies on the population of women with normal thyroid function, while the supplementation of women with synthetic levothyroxine did not affect the IQ of their children. The correlations of perchlorate levels in the body with other biochemical parameters are also o great interest. The work of Schreinemachers et al. [134] based on data obtained during NHANES 2001–2002 reports significant correlations between perchlorate and thiocyanate and nitrate and the level of hemoglobin (Hb), hematocrit (HCT) and the concentration of high density lipoproteins (HDL). In the sample examined, on the basis of the criteria adopted by the authors, there were 1,010 men and 1,084 women, the mean concentration of perchlorate in urine amounted to 5.99 and 5.03 μg/L, respectively. A significant negative correlation between urinary perchlorate concentration and level of Hb and HCT in the group of men, women aged 25–49 and pregnant women and for HDL only in the group of men were described. Another work by Schreinemachers et al. [135] advances the study of the correlation between perchlorate concentration in urine and various blood biochemical parameters, searching for connections between ClO4− and oxidative stress and the effect of these ions on iron homeostasis in blood plasma. Drawing on the set of data obtained during NHANES 2005–2008, the results of 3,705 men and 3,323 women were analyzed, of which 356 were pregnant. The median (range) concentration of perchlorate in the urine of patients amounted to, 4.28 (0.18–348) and 3.53 (0.12–74) mg/L, respectively. In such a large group, significant positive relationships between concentrations were described: ClO4− in the urine correlated with the level of urea nitrogen and the number of lymphocytes in the blood. Besides, a significant negative dependence on the level of iron correlated with uric acid in the plasma and the amount of red blood cells in the blood in men and non – pregnant women were noticed. Within the group of pregnant women, analogous relationships are only defined for the level of nitrogen in the blood and iron in the plasma.
There are some papers which investigate into the possible relationship between perchlorate exposure and other health disruptions. Liu et al. [136] suggested linking the increased risk of type 2 diabetes with increased exposure to perchlorate and other goitrogens. Formed upon data obtained from NHANES 2001–2014, 11,443 people were tested for perchlorate levels and then obtained data were correlated with prevalence of diabetes in studied group. The median perchlorate concentration in urine was 3.32 μg/L. The critical positive correlation between increasing concentration of ClO4− in urine and increased risk of diabetes has been depicted. Ko et al. [137] described a significant negative impact of perchlorate on the level of parathyroid hormone in women (sample of 4,265 people, mean ClO4− concentration in urine was 3.38 μg/L), Shiue [138], in turn, presented the negative effect of perchlorate on the sense of hearing, while Zhang et al. [139] indicated a possible, but not conclusively proven relationship between perchlorate in the urine correlated with some other factors like obesity, urinary iodine etc. and the occurrence of papillary thyroid cancer (PTC). Table 4. shows correlation studies between perchlorate exposure and plasma thyroid hormone levels.
Over the last 10 years, numerous studies also describe other possible perchlorate side effects than just thyroid effects. There are also works on the risk of diabetes, thyroid cancer, changes in blood morphology, parathyroid disorder or even hearing disorders [135], [136], [137], [138], [139], [140]. The latter are less frequent than those focusing on disruptions of thyroid hormone levels, which are often missed out in various studies. The last decade of research initiates a method of simultaneous determination of many goitrogens (especially thiocyanate and nitrate, but also phthalates, phenols and others). Since many cases of thyroid disfunctions are not correlated with perchlorate exposure, it seems to be a justified operation. Nonetheless, examining the cumulative impact of several different compounds, such relationships are described. The issue of exposure to goitrogens (including perchlorate in particular) becomes an important problem to be solved especially among those most vulnerable groups such as pregnant women and newborns. The conducted research suggests there is a iodine deficiency in pregnant women in some parts of Europe, which in the context of exposure to perchlorate might have a decisive impact on the abnormal development of the nervous system in their offspring [109]. Moreover, studies conducted by Taylor et al. [133] appear to confirm that the dependence of IQ decrease in three-year-old children was invariably upheld along with the increase in perchlorate in the urine of their mothers. Certainly, further studies and affirmations are required to describe the correlation of goitrogen exposure and the size of newborns, as also in this case, existing reports [124], [125], [126], [127] do not allow for the formulation of unambiguous conclusions. In addition to the studies presented in the present review, perchlorate in human urine have also been found in China [8], [140], in Haiti [141] and Kuwait [142] and in maternal milk in the USA [83], [143] and in human blood in China [144]. The increasing number of current perchlorate studies in various countries indicates that the perchlorate problem is still not fully understood and assessed. In the light of new facts about NIS stoichiometry and discovery of symporter allosteric modulation, perchlorate environmental contamination may be more dangerous than described so far. Significant reduction of iodine transport caused by ClO4− may have critical impact in long-term exposure on low doses of perchlorate [11]. The mentioned results have to be proven, but in the case of confirmation it seems crucial to revise perchlorate accepted level in drinking water and dietary products. As reported, world’s greatest perchlorate deposits are in Atacama, Chile and the risk of contamination of soils and plants is significant [145]. Many of foodstuffs, especially fruits are exported worldwide [9], however the number of research concerning perchlorate pollution in this country is limited [145].
Analysis of the results of query “perchlorate AND human AND health” in Web of Science browser in the years 2000–2009 and 2010–2020 provides some information of the current directions of perchlorate studies and those from the past decade. In the 2000–2009 there was a number of 75 records relevant to the query and the main scopes of interest were Toxicology (n=28), Environmental Sciences (n=25), Analytical Chemistry (n=11) and Occupational Health (n=10). In the 2010–2020 the number of relevant papers has increased to 111. We observed that area of concern in case of Occupational Health (n=10) and Analytical Chemistry (n=9) is nearly equal to those reported in previous period. Interestingly, there is increasing number of articles relevant to Environmental Sciences (n=40) and Environmental Engineering (n=14), moreover the number of marked as relevant to Food Science Technology (n=11) is doubled. The results may suggest that there are some gaps of knowledge in the perchlorate environmental occurrence and treatment or removal methods. The primary directions of perchlorate studies were of concern of those topics. Unexpectedly the number of toxicological studies has decreased by half. It may suggest, that the perchlorate mode-of-action is currently well-known, anyway the prevailing data [11] points some fields that needed to be better explained, like NIS chemistry and stoichiometry and its role in persistent exposure on subclinical doses of perchlorate. A comprehensive meta-analysis of the literature would be useful to better assess the directions and future remarks, without significant risk of bias. A comparison of selected scopes of interest of the perchlorate before 2010 and from 2010 to present has been shown in Figure 2.
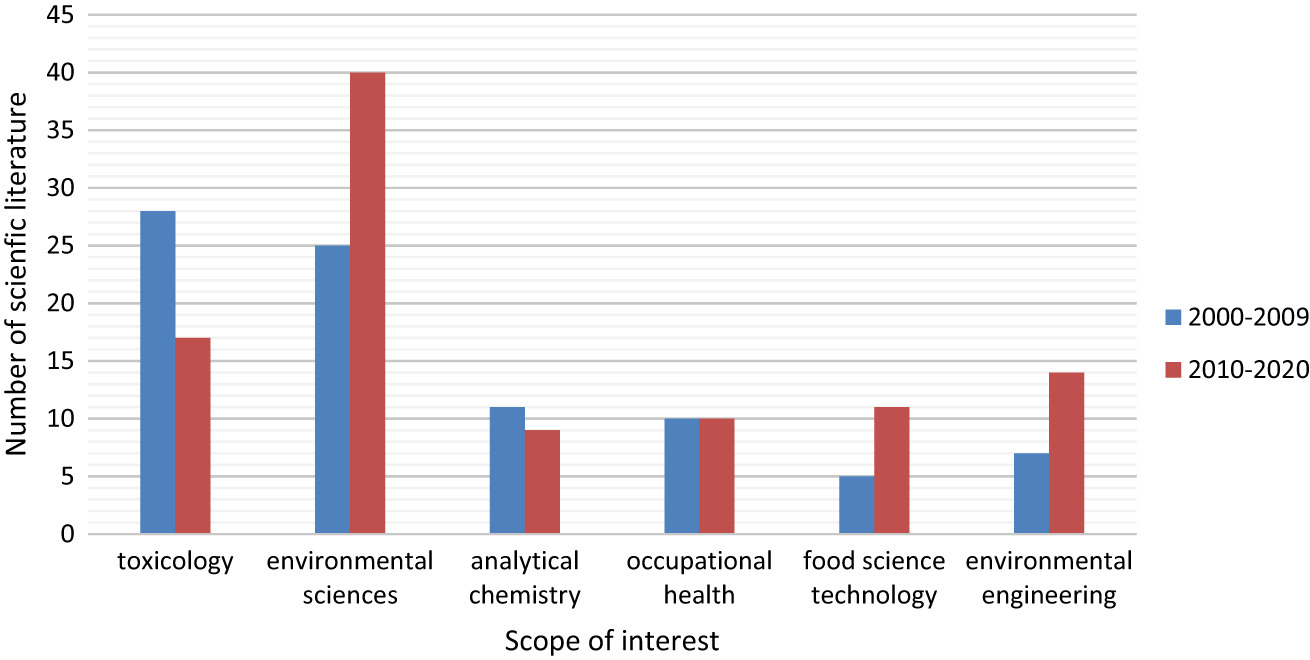
Comparison of perchlorate human health effects studies areas of concern in the years 2000–2009 and 2010–2020.
WHO estimates worldwide daily consumption of perchlorate on 0.03–0.22 μg bw/day, but this value may vary in certain geographical regions [146]. Even though the US National Academy of Sciences has established the perchlorate No-Observed-Adverse-Effect-Level (NOAEL) at 7 μg/kg of body weight/day and the reference dose for chronic oral perchlorate exposure of 0.7 μg/kg/bw [44] the research still yields conflicting results. What is more, the European Food Safety Authority (EFSA) in 2015 has published a report where tolerable daily intake were settled of 0.3 μg/kg/bw [92]. The NOAEL and reference dose were based on comprehensive and reliable study, but the estimation of this dose in children and pregnant woman turns out to be an elaborate and oftentimes unpredictable process. The NOAEL was settled after study on healthy male and female volunteers, but there is no evidence in specific subpopulations such as children and pregnant women [80]. It seems that despite the vast literature available on this matter, some problems still await further investigation. Crucially, perchlorate studies should lead to the settlement of reference dose for varying subpopulations, with regard to considering their specific metabolism. It appears that establishing specific NOAEL for each subpopulations of children, pregnant women, elders and people who suffer from various thyroid diseases is essential. Finally, re-evaluation of current NOAEL and reference dose and regulate perchlorate status and environmental monitoring in many countries may be useful for better protection of perchlorate exposure.
Conclusions
Despite more than 20 years of the described research conducted around the world, the problem of the impact of perchlorate on human health remains important and not fully comprehended. More than half of the works described in this review point to the existence of significant statistical relationship between the concentration of ClO4− in biological samples (mainly urine) and thyroid hormone levels, whereas the remaining studies present divergent conclusions. In this overview nearly 59% of works published between 2010 and 2020 found significant impact of perchlorate on human health. Having considered all the above-mentioned data, it is necessary to provide a large scale environmental monitoring, determining the levels of both perchlorate and other goitrogens. It is noticeable, that to our knowledge the number of the research literature concerning environmental and foodstuff occurrence of perchlorate nearly doubled in comparison to previous decade. In the light of evidence that the contribution of perchlorate from food products is several times higher than from drinking water countries shall implement continuous examination of dietary products. The implementation of new determination method that do not require expensive equipment would be useful in diffusing this idea worldwide.
Although EPA has recently reevaluated the available data on the frequency and level of perchlorate occurrence in the US public water systems, and concluded that there is infrequent occurrence of perchlorate at the levels of public health concern [148], such data is lacking in many parts of the world, so the issue is still relevant.
Our analysis of scientific databases provides an information that interest of perchlorate human health effects is still increasing, thus perchlorate pollution is still relevant and prevailing topic. Furthermore, to minimize the risk of exposure and related health effects it is essential to conduct human population studies on health effects of perchlorate in various parts of the world.
Research funding: None declared.
Author contributions: All authors have accepted responsibility for the entire content of this manuscript and approved its submission.
Competing interests: The authors declare they have no actual or potential competing financial interests.
Informed consent: Not applicable.
Ethical statements: Not applicable.
References
1. Urbansky, ET. Perchlorate as an environmental contaminant. Environ Sci Pollut Res 2002;9:187–92. https://doi.org/10.1007/BF02987487.Search in Google Scholar PubMed
2. Dasgupta, PK, Martinelango, PK, Jackson, WA, Anderson, TA, Tian, K, Tock, RW, et al. The origin of naturally occurring perchlorate: the role of atmospheric processes. Environ Sci Technol 2005;39:1569–75. https://doi.org/10.1021/es048612x.Search in Google Scholar PubMed
3. Soldin, OP, Braverman, LE, Lamm, SH. Perchlorate clinical pharmacology and human health: a review. Ther Drug Monit 2001;23:316–31. https://doi.org/10.1080/10810730902873927.Testing.Search in Google Scholar
4. Urbansky, ET, Schock, MR. Issues in managing the risks associated with perchlorate in drinking water. J Environ Manag 1999;56:79–95. https://doi.org/10.1006/jema.1999.0274.Search in Google Scholar
5. Gibbs, JP, Ahmad, R, Crump, KS, Houck, DP, Leveille, TS, Findley, JE, et al. Evaluation of a population with occupational exposure to airborne ammonium perchlorate for possible acute or chronic effects on thyroid function. J Occup Environ Med 1998;40:1072–82. https://doi.org/10.1097/00043764-199812000-00007.Search in Google Scholar PubMed
6. ATSDR. Toxicological profile for perchlorates. Atlanta, GA; 2008.Search in Google Scholar
7. Zhang, T, Chen, X, Wang, D, Li, R, Ma, Y, Mo, W, et al. Perchlorate in indoor dust and human urine in China: contribution of indoor dust to total daily intake. Environ Sci Technol 2015;49:2443–50. https://doi.org/10.1021/es504444e.Search in Google Scholar PubMed
8. Kumarathilaka, P, Oze, C, Indraratne, SP, Vithanage, M. Perchlorate as an emerging contaminant in soil, water and food. Chemosphere 2016;150:667–77. https://doi.org/10.1016/J.CHEMOSPHERE.2016.01.109.Search in Google Scholar PubMed
9. Calderon, R, Godoy, F, Escudey, M, Palma, P. A review of perchlorate (ClO4−) occurrence in fruits and vegetables. Environ Monit Assess 2017;189. https://doi.org/10.1007/s10661-017-5793-x.Search in Google Scholar PubMed
10. Tarone, RE, Lipworth, L, McLaughlin, JK. The epidemiology of environmental perchlorate exposure and thyroid function: a comprehensive review. J Occup Environ Med 2010;52:653–60.10.1097/JOM.0b013e3181e31955Search in Google Scholar PubMed
11. Llorente-Esteban, A, Manville, RW, Reyna-Neyra, A, Abbott, GW, Amzel, LM, Carrasco, N, et al. Allosteric regulation of mammalian Na+/I− symporter activity by perchlorate. Nat Struct Mol Biol 2020;27:533–9. https://doi.org/10.1038/s41594-020-0417-5.Search in Google Scholar PubMed
12. Mattie, DR, Strawson, J, Zhao, J. Perchlorate toxicity and risk assessment. In: Perchlorate: environmental occurrence, interactions and treatment. Springer US; 2006:pp. 169–96. https://doi.org/10.1007/0-387-31113-0_8.Search in Google Scholar
13. Von Stadion, F. Von den Verbindungen der Chlorine mit dem Sauerstoff. Ann Phys 1816;52:219.10.1002/andp.18160520204Search in Google Scholar
14. Schilt, AA. Perchloric acid and perchlorates. G. F. Smith Chemical Company; 1979. Available from: https://books.google.pl/books?id=XKN8QgAACAAJ.Search in Google Scholar
15. Dasgupta, PK, Dyke, J V., Kirk, AB, Jackson, WA. Perchlorate in the United States. Analysis of relative source contributions to the food chain. Environ Sci Technol 2006;40:6608–14. https://doi.org/10.1021/es061321z.Search in Google Scholar PubMed
16. Trumpolt, CW, Crain, M, Cullison, GD, Flanagan, SJP, Siegel, L, Lathrop, S. Perchlorate: sources, uses, and occurrences in the environment. Remediation 2005;16:65–89. https://doi.org/10.1002/rem.20071.Search in Google Scholar
17. Kociołek-Balawejder, E, Wilk, Ł. Nadchlorany - nowe mikrozanieczyszczenie środowiska naturalnego. Pr Nauk Uniw Ekon we Wrocławiu Nauk Inżynierskie i Technol 2009;57:216–29.Search in Google Scholar
18. Susarla, S, Collette, TW, Garrison, AW, Wolfe, NL, Mccutcheon, SC. Perchlorate identification in fertilizers. Environ Sci Technol 1999;33:3469–72. https://doi.org/10.1021/es990577k.Search in Google Scholar
19. Urbansky, ET, Brown, SK. Perchlorate retention and mobility in soils. J Environ Monit 2003;5:455–62. https://doi.org/10.1039/B301125A.Search in Google Scholar PubMed
20. United Nations Trade Statistics Branch. Available from: https://comtrade.un.org/data/ [Accessed 7 Oct 2019].Search in Google Scholar
21. Du, Z, Xiao, C, Furdui, VI, Zhang, W. The perchlorate record during 1956-2004 from Tienshan ice core, East Asia. Sci Total Environ 2019;656:1121–32 https://doi.org/10.1016/j.scitotenv.2018.11.456.Search in Google Scholar PubMed
22. Rao, B, Anderson, TA, Orris, GJ, Rainwater, KA, Rajagopalan, S, Sandvig, RM, et al. Widespread natural perchlorate in unsaturated zones of the southwest United States. Environ Sci Technol 2007;41:4522–8. https://doi.org/10.1021/es062853i.Search in Google Scholar PubMed
23. Kounaves, SP, Stroble, ST, Anderson, RM, Moore, Q, Catling, DC, Douglas, S, et al. Discovery of natural perchlorate in the antarctic dry valleys and its global implications. Environ Sci Technol 2010;44:2360–4. https://doi.org/10.1021/es9033606.Search in Google Scholar PubMed
24. Poghosyan, A, Sturchio, NC, Morrison, CG, Beloso, AD, Guan, Y, Eiler, JM, et al. Perchlorate in the great Lakes: isotopic composition and origin. Environ Sci Technol 2014;48:11146–53. https://doi.org/10.1021/es502796d.Search in Google Scholar PubMed
25. Van Stempvoort, DR, Struger, J, Brown, SJ. Perchlorate in environmental waters of the Laurentian Great Lakes watershed: evidence for uneven loading. J Great Lake Res 2019;45:240–51. https://doi.org/10.1016/j.jglr.2018.12.007.Search in Google Scholar
26. Jackson, A, Davila, AF, Böhlke, JK, Sturchio, NC, Sevanthi, R, Estrada, N, et al. Deposition, accumulation, and alteration of Cl-, NO3-, ClO4-and ClO3-salts in a hyper-arid polar environment: mass balance and isotopic constraints. Geochem Cosmochim Acta 2016;182:197–215. https://doi.org/10.1016/j.gca.2016.03.012.Search in Google Scholar
27. Simonaitis, R, Heicklen, J. Perchloric acid: a possible sink for stratospheric chlorine. Planet Space Sci 1975;23:1567–9. https://doi.org/10.1016/0032-0633(75)90010-0.Search in Google Scholar
28. Jaegle, L, Yung, YL, Toon, GC, Sen, B, Blavier, JF. Balloon observations of organic and inorganic chlorine in the stratosphere: the role of HClO4 production on sulfate aerosols. Geophys Res Lett 1996;23:1749–52. https://doi.org/10.1029/96GL01543.Search in Google Scholar
29. Böhlke, JK, Ericksen, GE, Revesz, K. Stable isotope evidence for an atmospheric origin of desert nitrate deposits in northern Chile and southern California. U.S.A. Chem Geol. 1997;136:135–52. https://doi.org/10.1016/S0009-2541(96)00124-6.Search in Google Scholar
30. Michalski, G, Böhlke, JK, Thiemens, M. Long term atmospheric deposition as the source of nitrate and other salts in the Atacama Desert, Chile: new evidence from mass-independent oxygen isotopic compositions. Geochem Cosmochim Acta 2004;68:4023–38. https://doi.org/10.1016/j.gca.2004.04.009.Search in Google Scholar
31. Catling, DC, Claire, MW, Zahnle, KJ, Quinn, RC, Clark, BC, Hecht, MH, et al. Atmospheric origins of perchlorate on mars and in the atacama. J Geophys Res E Planets 2010;115:2018. https://doi.org/10.1029/2009JE003425.Search in Google Scholar
32. Fang, Q, Chen, B. Natural origins, formation mechanisms, and fate of environmental perchlorate. Prog Chem 2012;24:2040–53.Search in Google Scholar
33. Kang, N, Jackson, WA, Dasgupta, PK, Anderson, TA. Perchlorate production by ozone oxidation of chloride in aqueous and dry systems. Sci Total Environ 2008;405:301–9. https://doi.org/10.1016/j.scitotenv.2008.07.010.Search in Google Scholar PubMed
34. Jackson, WA, Rainwater, KA, Anderson, TA, Lehman, T. Distribution and potential sources of perchlorate in the high plains region of Texas. Final Report Submitt to Texas Comm Environ Qual Austin;2004 (January 2003).Search in Google Scholar
35. Jackson, WA, Gu, B, Heikoop, JM. Chlorine-36 as a tracer of perchlorate origin. Environ Sci Technol 2009;43:6934–8. https://doi.org/10.1021/es9012195.Search in Google Scholar PubMed
36. SERDP. Alternative causes of wide-spread, low concentration perchlorate impacts to groundwater. SERDP, Arlington, VA; 2005.Search in Google Scholar
37. Gu, B, Coates, JD. Perchlorate: environmental occurrence, interactions and treatment. Boston, MA: Springer; 2006. https://doi.org/10.1007/0-387-31113-0.Search in Google Scholar
38. Cotton, FA, Geoffrey, W. Advanced inorganic chemistry: a comprehensive text, 3rd ed. New York, NY: John Wiley & Sons; 1972.Search in Google Scholar
39. Lee, FS, Carpenter, GB. The crystal structure of perchloric acid monohydrate. J Phys Chem 1959;63:279–83. https://doi.org/10.1021/j150572a035.Search in Google Scholar
40. Simon, A, Borrmann, H. The structures of perchloric acid, HClO4, and its anhydride, Cl2O7. Angew Chem Int Ed Engl 1988;27:1339–41. https://doi.org/10.1002/anie.198813391.Search in Google Scholar
41. Yang, Y. Recent advances in the electrochemical oxidation water treatment: spotlight on byproduct control. Front Environ Sci Eng 2020;14:85 https://doi.org/10.1007/s11783-020-1264-7.Search in Google Scholar
42. Wu, Q, Zhang, T, Sun, H, Kannan, K. Perchlorate in tap water, groundwater, surface waters, and bottled water from China and its association with other inorganic anions and with disinfection byproducts. Arch Environ Contam Toxicol 2010;58:543–50. https://doi.org/10.1007/s00244-010-9485-6.Search in Google Scholar PubMed
43. Bergmann, H, Iourtchouk, T, Schmidt, W, Nüske, G. Perchlorate formation in electrochemical water disinfection. Hauppauge, NY: Nova Science Publishers; 2011.Search in Google Scholar
44. National Research Council. Health implications of perchlorate ingestion. Washington, DC: National Academies Press; 2005. https://doi.org/10.17226/11202.Search in Google Scholar
45. Dotson, RL, Ralston, RW, Loftis, HJ. Process for producting perchloric acid and ammonium perchlorate. US patent; 1992.Search in Google Scholar
46. Dotson, RL. A novel electrochemical process for the production of ammonium perchlorate. J Appl Electrochem 1993;23:897–904. https://doi.org/10.1007/BF00251024.Search in Google Scholar
47. Welcher, FJ. Organic analytical reagents. London: D. Van Nostrand Company; 1947. https://doi.org/10.1021/j150454a029.Search in Google Scholar
48. Harris, D. Quantitative chemical analysis, 3rd ed. New York, NY: Freeman; 1991.Search in Google Scholar
49. Lamb, A, Marden, J. The quantitative determination of perchlorates. J Am Chem Soc 1912;34:812–17.10.1021/ja02207a008Search in Google Scholar
50. Nabar, GM, Ramachandran, CR. Quantitative determination of perchlorate ion in solution. Anal Chem 1959;31:263–5. https://doi.org/10.1021/ac60146a033.Search in Google Scholar
51. Zatko, DA, Kratochvil, B. Vanadium(III) sulfate as a reducing agent for determination of perchlorate. Anal Chem 1965;37:1560–2. https://doi.org/10.1021/ac60231a026.Search in Google Scholar
52. Fogg, AG, Burns, DT, Yeowart, EH. Determination of perchlorate in samples of potassium chlorate. II. Microchim Acta 1970;58:974–7. https://doi.org/10.1007/BF01225728.Search in Google Scholar
53. Tsubouchi, M. Spectrophotometric determination of anions by solvent extraction with neutral red. Anal Chim Acta 1971;54:143–8. https://doi.org/10.1016/S0003-2670(01)81864-X.Search in Google Scholar
54. Burns, DT, Tungkananuruk, N. Spectrophotometric determination of perchlorate after extraction of its brilliant green ion-pair with microcrystalline benzophenone. Anal Chim Acta 1987;199:237–40. https://doi.org/10.1016/S0003-2670(00)82822-6.Search in Google Scholar
55. Burns, DT, Dunford, M., Sutthivaiyakit, P. Spectrophotometric determination of perchlorate after extraction as protriptylinium perchlorate. Anal Chim Acta 1997;356:141–3. https://doi.org/10.1016/S0003-2670(97)00445-5.Search in Google Scholar
56. Burns, EA, Muraca, RF. Volumetric assay of ammonium perchlorate. Anal Chem 1960;32:1316–19. https://doi.org/10.1021/ac60166a025.Search in Google Scholar
57. Aravamudan, G, Krishnan, V. A new method for the titrimetric determination of perchlorate. Talanta 1966;13:519–22. https://doi.org/10.1016/0039-9140(66)80072-3.Search in Google Scholar
58. Shahine, S, Ismael, N. Indirect bromometric microdetermination of nitrate and perchlorate, using nitron as reagent. Microchim Acta 1976;66:75–9. https://doi.org/10.1007/BF01257096.Search in Google Scholar
59. Kim, Y, Amemiya, S. Stripping analysis of nanomolar perchlorate in drinking water with a voltammetric ion-selective electrode based on thin-layer liquid membrane. Anal Chem 2008;80:6056–65. https://doi.org/10.1021/ac8008687.Search in Google Scholar
60. Goncharuk, VV., Zui, OV., Kushchevskaya, NF. Methods of determining perchlorates. J Water Chem Technol 2009;31:186–94. https://doi.org/10.3103/S1063455X09030072.Search in Google Scholar
61. Biesaga, M, Kwiatkowska, M, Trojanowicz, M. Separation of chlorine-containing anions by ion chromatography and capillary electrophoresis. J Chromatogr A 1997;777:375–81. https://doi.org/10.1016/S0021-9673(97)00338-5.Search in Google Scholar
62. California Department of Health Services. Determination of perchlorate by ion chromatography. Rev. 0; 1997. Sanitation and Radiation Laboratories Branch.Search in Google Scholar
63. Wagner, HP, Pepich, B V, Pohl, C, Later, D, Srinivasan, K, Lin, R, et al. Selective method for the analysis of perchlorate in drinking waters at nanogram per liter levels , using two-dimensional ion chromatography with suppressed conductivity detection. J Chromatogr A 2007;1155:15–21. https://doi.org/10.1016/j.chroma.2007.03.025.Search in Google Scholar PubMed
64. Michalski, R, Łyko, A, Kernert, J. Chlorany(VII) w środowisku – problemy i wyzwania. In: Michalski, R, editor Chromatografia Jonowa 2013. Zabrze: IPIŚ PAN; 2013:pp. 24–42.10.1016/j.orgdyn.2012.12.005Search in Google Scholar
65. Hautman, DP, Munch, DJ. Determination of perchlorate in drinking water using ion. OH: Cincinanati; 1999.Search in Google Scholar
66. Wagner, HP, Pepich, B V, Pohl, C, Later, D, Joyce, R, Srinivasan, K, et al. US Environmental Protection Agency Method 314 . 1 , an automated sample preconcentration / matrix elimination suppressed conductivity method for the analysis of trace levels (0 . 50 µg / L) of perchlorate in drinking water. J Chromatogr A 2006;1118:85–93. https://doi.org/10.1016/j.chroma.2006.02.039.Search in Google Scholar PubMed
67. Wendelken, S, Munch, D, Pepich, B, Later, D, Pohl, C. Determination of perchlorate in drinking water by liquid chromatography electrospray ionization mass spectrometry. OH: Cincinanati; 2005.Search in Google Scholar
68. Hendrick, E, Behymer, T, Slingsby, R, Munch, D. Determination of perchlorate in drinking water by ion chromatography with suppressed conductivity and electrospray ionization mass spectrometry. OH: Cincinanati; 2005.Search in Google Scholar
69. El Aribi, H, Le Blanc, YJC, Antonsen, S, Sakuma, T. Analysis of perchlorate in foods and beverages by ion chromatography coupled with tandem mass spectrometry (IC-ESI-MS/MS). Anal Chim Acta 2006;567:39–47. https://doi.org/10.1016/j.aca.2006.03.012.Search in Google Scholar PubMed
70. Luis, SJ, Miesner, EA, Enslin, CL, Heidecorn, K. Review of perchlorate occurrence in large public drinking water systems in the United States of America. Water Sci Technol Water Supply 2019;19:681–94. https://doi.org/10.2166/ws.2018.135.Search in Google Scholar
71. Liu, W, Lian, J, Guo, J, Zhang, C, Guo, Y, Niu, Y, et al. Perchlorate reduction by anaerobic granular sludge under different operation strategies: performance, extracellular polymeric substances and microbial community. Bioresour Technol Rep 2019;8:100312. https://doi.org/10.1016/j.biteb.2019.100312.Search in Google Scholar
72. Dong, H, Atlas, E, Wade, MG. Development of a non-radioactive screening assay to detect chemicals disrupting the human sodium iodide symporter activity. Toxicol Vitro 2019;57:39–47. https://doi.org/10.1016/j.tiv.2019.01.021.Search in Google Scholar PubMed
73. Liao, Z, Cao, D, Gao, Z, Zhang, S. Occurrence of perchlorate in processed foods manufactured in China. Food Contr 2020;107. https://doi.org/10.1016/j.foodcont.2019.106813.Search in Google Scholar
74. Bardiya, N, Bae, JH. Dissimilatory perchlorate reduction: a review. Microbiol Res 2011;166:237–54. https://doi.org/10.1016/j.micres.2010.11.005.Search in Google Scholar PubMed
75. Srinivasan, A, Viraraghavan, T. Perchlorate: health effects and technologies for its removal from water resources. Int J Environ Res Publ Health 2009;6:1418–42. https://doi.org/10.3390/ijerph6041418.Search in Google Scholar PubMed PubMed Central
76. Lamm, S, Braverman, E.L, Xiao Li, F, Richman, K, Pino, S, Howearth, G. Thyroid health status of ammonium perchlorate workers: a cross-sectional occupational health study. J Occup Environ Med 1999;41:248–60. https://doi.org/10.1097/00043764-199904000-00006.Search in Google Scholar PubMed
77. Chen, H, Wu, L, Wang, X, Liu, Q, Ding, M, Peng, K, et al. Perchlorate exposure and thyroid function in ammonium perchlorate workers in Yicheng, China. Int J Environ Res Publ Health 2014;11:4926–38. https://doi.org/10.3390/ijerph110504926.Search in Google Scholar PubMed PubMed Central
78. Zhang, T, Chen, X, Wang, D, Li, R, Ma, Y, Mo, W, et al. Perchlorate in indoor dust and human urine in China: contribution of indoor dust to total daily intake. Environ Sci Technol 2015;49:2443–50. https://doi.org/10.1021/es504444e.Search in Google Scholar PubMed
79. Snyder, SA, Pleus, RC, Vanderford, BJ, Holady, JC. Perchlorate and chlorate in dietary supplements and flavor enhancing ingredients. Anal Chim Acta 2006;567:26–32. https://doi.org/10.1016/j.aca.2006.03.029.Search in Google Scholar PubMed
80. Greer, M, Gooodman, G, Richard, P, Greer, S. Health effects assessment for environmental perchlorate contamination: the dose response for inhibition of thyroidal radioiodine uptake in humans. Environ Health Perspect 2002;110:927–37. https://doi.org/10.1289/ehp.02110927.Search in Google Scholar PubMed PubMed Central
81. U.S. Environmental Protection Agency. 2012 Edition of the drinking water standards and health advisories. Washington, DC: U.S. Environmental Protection Agency; 2012.Search in Google Scholar
82. Isanhart, JP, McNabb, FMA, Smith, PN. Effects of perchlorate exposure on resting metabolism, peak metabolism, and thyroid function in the prairie vole (Microtus ochrogaster). Environ Toxicol Chem 2005;24:678–84.10.1897/04-306R.1Search in Google Scholar PubMed
83. Dasgupta, PK, Kirk, AB, Dyke, J V., Ohira, SI. Intake of iodine and perchlorate and excretion in human milk. Environ Sci Technol 2008;42:8115–21. https://doi.org/10.1021/es801549w.Search in Google Scholar PubMed
84. Brandhuber, P, Clark, S, Morley, K. A review of perchlorate occurrence in public drinking water systems. J AWWA (Am Water Works Assoc) 2009;101:63–73. https://doi.org/10.1002/j.1551-8833.2009.tb09991.x.Search in Google Scholar
85. Cao, F, Jaunat, J, Sturchio, N, Cancès, B, Morvan, X, Devos, A, et al. Worldwide occurrence and origin of perchlorate ion in waters: a review. Sci Total Environ 2019;661:737–49. https://doi.org/10.1016/j.scitotenv.2019.01.107.Search in Google Scholar PubMed
86. Sijimol, MR, Mohan, M, Dineep, D. Perchlorate contamination in bottled and other drinking water sources of Kerala, southwest coast of India. Energy, Ecol Environ 2016;1:148–56. https://doi.org/10.1007/s40974-016-0018-7.Search in Google Scholar
87. Calderón, R, Palma, P, Parker, D, Escudey, M. Capture and accumulation of perchlorate in lettuce. Effect of genotype, temperature, perchlorate concentration, and competition with anions. Chemosphere 2014;111:195–200. https://doi.org/10.1016/j.chemosphere.2014.03.027.Search in Google Scholar PubMed
88. Calderon, R, Rajendiran, K, Kim, UJ, Palma, P, Miranda, NA, Moreno, ES, et al. Sources and fates of perchlorate in soils in Chile: a case study of perchlorate dynamics in soil-crop systems using lettuce (Lactuca sativa) fields. Environ Pollut 2020;264:114682. https://doi.org/10.1016/j.envpol.2020.114682.Search in Google Scholar PubMed
89. Wang, Z, Forsyth, D, Lau, BP-Y, Pelletier, L, Bronson, R, Gaertner, D, et al. Estimated dietary exposure of Canadians to perchlorate through the consumption of fruits and vegetables available in ottawa markets. J Agric Food Chem 2009;57:9250–5. https://doi.org/10.1021/jf901910x.Search in Google Scholar PubMed
90. Lee, JW, Oh, SH, Oh, JE. Monitoring of perchlorate in diverse foods and its estimated dietary exposure for Korea populations. J Hazard Mater 2012;243:52–8. https://doi.org/10.1016/j.jhazmat.2012.09.037.Search in Google Scholar PubMed
91. Liao, Z, Cao, D, Gao, Z, Zhang, S. Occurrence of perchlorate in processed foods manufactured in China. Epub ahead of print 1 January. Food Contr 2020;107. https://doi.org/10.1016/j.foodcont.2019.106813.Search in Google Scholar
92. EFSA Panel on Contaminants in the Food Chain (CONTAM). Scientific Opinion on the risks to public health related to the presence of perchlorate in food, in particular fruits and vegetables. EFSA J 2014;12:3869. https://doi.org/10.2903/j.efsa.2014.3869.Search in Google Scholar
93. Huber, D, Blount, B, Mage, D, Letkiewicz, FJ, Kumar, A, Allen, RH, et al. Estimating perchlorate exposure from food and tap water based on US biomonitoring and occurrence data. J Expo Sci Environ Epidemiol 2011;21:395–407. https://doi.org/10.1038/jes.2010.31.Search in Google Scholar PubMed
94. Arcella, D, Binaglia, M, Vernazza, F. Dietary exposure assessment to perchlorate in the European population. Epub ahead of print. EFSA J 2017;15. https://doi.org/10.2903/j.efsa.2017.5043.Search in Google Scholar PubMed PubMed Central
95. European Comission. COMMISSION REGULATION (EU) 2020/685 of 20 May 2020 amending Regulation (EC) No 1881/2006 as regards maximum levels of perchlorate in certain foods. Official Journal of the European Union; 2020.Search in Google Scholar
96. Smanik, PA, Liu, Q, Furminger, TL, Ryu, K, Xing, S, Mazzafferi, EL, et al. Cloning of the human sodium iodide symporter. Biochem Biophys Res Commun 1996;226:339–45. https://doi.org/10.1006/bbrc.1996.1358.Search in Google Scholar PubMed
97. Wolff, J. Perchlorate and the thyroid gland. Pharmacol Rev 1998;50:89–105.Search in Google Scholar
98. Eskandari, S, Loo, DDF, Dai, G, Levy, O, Wright, EM, Carrasco, N. Thyroid Na+ /I − symporter. Mechanism, stoichiometry, and specificity. J Biol Chem 1997;272:27230–8.10.1074/jbc.272.43.27230Search in Google Scholar PubMed
99. Dohán, O, Portulano, C, Basquin, C, Reyna-Neyra, A, Amzel, LM, Carrasco, N, et al. The Na+/I- symporter (NIS) mediates electroneutral active transport of the environmental pollutant perchlorate. Proc Natl Acad Sci Unit States Am 2007; 104: 20250–255. https://doi.org/10.1073/pnas.0707207104.Search in Google Scholar PubMed PubMed Central
100. Błażewicz, A, Makarewicz, A, Korona-Glowniak, I, Dolliver, W, Kocjan, R. Iodine in autism spectrum disorders. J Trace Elem Med Biol 2016;34:32–7. https://doi.org/10.1016/j.jtemb.2015.12.002.Search in Google Scholar PubMed
101. Blaurock-Busch, E, Amin, OR, Dessoki, HH, Rabah, T. Toxic metals and essential elements in hair and severity of symptoms among children with autism. Maedica (Buchar). 2012;7:38–48.Search in Google Scholar
102. Spitzweg, C, Joba, W, Schriever, K, Goellner, JR. Analysis of human sodium iodide symporter immunoreactivity in human exocrine Glands1. J Clin Endocrinol Metab 1999;84:4178–84. https://doi.org/10.1210/jc.84.11.4178.Search in Google Scholar
103. Johnson, RS, Moore, WG. Fatal aplastic anaemia after treatment of thyrotoxicosis with potassium perchlorate. Br Med J 1961;1:1369–71. https://doi.org/10.1136/bmj.1.5236.1369.Search in Google Scholar PubMed PubMed Central
104. Hobson, QT. Aplastic anaemia due to treatment with potassium perchlorate. Br Med J 1961;1:1368–9. https://doi.org/10.1136/bmj.1.5236.1368.Search in Google Scholar PubMed PubMed Central
105. Krevans, JR, Asper, SPJ, Rienhoff, WFJ. Fatal aplastic anemia following use of potassium perchlorate in thyrotoxicosis. J Am Med Assoc 1962;181:162–4. https://doi.org/10.1001/jama.1962.03050280092013c.Search in Google Scholar PubMed
106. Gjemdal, N. Fatal aplastic anaemia following use of potassium perchlorate in thyrotoxicosis. Acta Med Scand 1963;174:129–31. https://doi.org/10.1111/j.0954-6820.1963.tb07902.x.Search in Google Scholar PubMed
107. Barzilai, D, Sheinfeld, M. Fatal complications following use of potassium perchlorate in thyrotoxicosis. Report of two cases and a review of the literature. Isr J Med Sci 1966;2:453–6. https://doi.org/10.1111/j.0954-6820.1963.tb07902.x.Search in Google Scholar
108. Liberati, A, Altman, DG, Tetzlaff, J, Mulrow, C, Gøtzsche, PC, Ioannidis, JPA, et al. The PRISMA statement for reporting systematic reviews and meta-analyses of studies that evaluate health care interventions: explanation and elaboration. PLoS Med 2009;6. https://doi.org/10.1371/journal.pmed.1000100.Search in Google Scholar PubMed PubMed Central
109. Pearce, EN, Lazarus, JH, Smyth, PPA, He, X, Dall’Amico, D, Parkes, AB, et al. Perchlorate and thiocyanate exposure and thyroid function in first-trimester pregnant women. J Clin Endocrinol Metab 2010;95:3207–15. https://doi.org/10.1210/jc.2010-0014.Search in Google Scholar PubMed
110. Pearce, E, Spencer, C, Mestman, J, Lee, R, Bergoglio, L, Mereshian, P, et al. Effect of environmental perchlorate on thyroid function in pregnant women from córdoba, Argentina, and Los Angeles, California. Endocr Pract 2011. https://doi.org/10.4158/EP10293.OR.Search in Google Scholar PubMed
111. Pearce, EN, Alexiou, M, Koukkou, E, Braverman, LE, He, X, Ilias, I, et al. Perchlorate and thiocyanate exposure and thyroid function in first-trimester pregnant women from Greece. Clin Endocrinol 2012;77:471–4. https://doi.org/10.1111/j.1365-2265.2012.04407.x.Search in Google Scholar PubMed
112. Valentin-Blasini, L, Blount, BC, Delinsky, A. Quantification of iodide and sodium-iodide symporter inhibitors in human urine using ion chromatography tandem mass spectrometry. J Chromatogr A 2007;1155:40–6. https://doi.org/10.1016/j.chroma.2007.04.014.Search in Google Scholar PubMed
113. Leung, AM, Braverman, LE, He, X, Schuller, KE, Roussilhes, A, Jahreis, K, et al. Environmental perchlorate and thiocyanate exposures and infant serum thyroid function. Thyroid 2012;22:938–43. https://doi.org/10.2478/hukin-2013-0026.Search in Google Scholar PubMed PubMed Central
114. Mortensen, ME, Birch, R, Wong, LY, Valentin-Blasini, L, Boyle, EB, Caldwell, KL, et al. Thyroid antagonists and thyroid indicators in U.S. pregnant women in the Vanguard Study of the National Children’s Study. Environ Res 2016. https://doi.org/10.1016/j.envres.2016.05.017.Search in Google Scholar PubMed PubMed Central
115. Gold, EB, Blount, BC, O’neill Rasor, M, Lee, JS, Alwis, U, Srivastav, A. Thyroid hormones and thyroid disease in relation to perchlorate dose and residence near a superfund site. J Expo Sci Environ Epidemiol 2013;23:399–408. https://doi.org/10.1038/jes.2012.90.Search in Google Scholar PubMed PubMed Central
116. Ucal, Y, Sahin, ON, Serdar, M, Blount, B, Kumru, P, Muhcu, M, et al. Exposure to perchlorate in lactating women and its associations with newborn thyroid stimulating hormone. Front Endocrinol 2018;9:348. https://doi.org/10.3389/fendo.2018.00348.Search in Google Scholar PubMed PubMed Central
117. Mendez, W, Eftim, SE. Biomarkers of perchlorate exposure are correlated with circulating thyroid hormone levels in the 2007-2008 NHANES. Environ Res 2012;118:137–44. https://doi.org/10.1016/j.envres.2012.05.010.Search in Google Scholar PubMed
118. Steinmaus, C, Miller, MD, Cushing, L, Blount, BC, Smith, AH. Combined effects of perchlorate, thiocyanate, and iodine on thyroid function in the national health and nutrition examination survey 2007-8. Environ Res. 2013;123:1–27. https://doi.org/10.1016/j.envres.2013.01.005.Search in Google Scholar PubMed PubMed Central
119. Przybyla, J, Geldhof, GJ, Smit, E, Kile, ML. A cross sectional study of urinary phthalates, phenols and perchlorate on thyroid hormones in US adults using structural equation models (NHANES 2007–2008). Environ Res 2018;163:26–35. https://doi.org/10.1016/j.envres.2018.01.039.Search in Google Scholar PubMed PubMed Central
120. Charatcharoenwitthaya, N, Ongphiphadhanakul, B, Pearce, EN, Somprasit, C, Chanthasenanont, A, He, X, et al. The association between perchlorate and thiocyanate exposure and thyroid function in first-trimester pregnant Thai women. J Clin Endocrinol Metab 2014;99:2365–71. https://doi.org/10.1210/jc.2013-3986.Search in Google Scholar PubMed
121. Horton, MK, Blount, BC, Valentin-Blasini, L, Wapner, R, Whyatt, R, Gennings, C, et al. Co-occurring exposure to perchlorate, nitrate and thiocyanate alters thyroid function in healthy pregnant women. Environ Res 2015;143:1–9. https://doi.org/10.1016/j.envres.2015.09.013.Search in Google Scholar PubMed PubMed Central
122. Steinmaus, C, Pearl, M, Kharrazi, M, Blount, BC, Miller, MD, Pearce, EN, et al. Thyroid hormones and moderate exposure to perchlorate during pregnancy in women in southern California. Environ Health Perspect 2016;124:861–7. https://doi.org/10.1289/ehp.1409614.Search in Google Scholar PubMed PubMed Central
123. Cao, Y, Blount, BC, Valentin-Blasini, L, Bernbaum, JC, Phillips, TM, Rogan, WJ. Goitrogenic anions, thyroid-stimulating hormone, and thyroid hormone in infants. Environ Health Perspect 2010;118:1332–7. https://doi.org/10.1289/ehp.0901736.Search in Google Scholar PubMed PubMed Central
124. Knight, BA, Shields, BM, He, X, Pearce, EN, Braverman, LE, Sturley, R, et al. Effect of perchlorate and thiocyanate exposure on thyroid function of pregnant women from South-West England: a cohort study. Thyroid Res 2018;11:9. https://doi.org/10.1186/s13044-018-0053-x.Search in Google Scholar PubMed PubMed Central
125. Rubin, R, Pearl, M, Kharrazi, M, Blount, BC, Miller, MD, Pearce, EN, et al. Maternal perchlorate exposure in pregnancy and altered birth outcomes. Environ Res 2017;158:72–7.81 https://doi.org/10.1016/j.envres.2017.05.030.Search in Google Scholar PubMed PubMed Central
126. Evans, KA, Rich, DQ, Weinberger, B, Vetrano, AM, Blasini, LV, Strickland, PO, et al. Association of prenatal perchlorate, thiocyanate, and nitrate exposure with neonatal size and gestational age. Reprod Toxicol 2015;57:183–7. https://doi.org/10.1016/j.reprotox.2015.07.069.Search in Google Scholar PubMed PubMed Central
127. Blount, BC, Rich, DQ, Valentin-Blasini, L, Lashley, S, Ananth, CV, Murphy, E, et al. Perinatal exposure to perchlorate, thiocyanate, and nitrate in New Jersey mothers and newborns. Environ Sci Technol 2009;43:7543–9. https://doi.org/10.1021/es9008486.Search in Google Scholar PubMed PubMed Central
128. Mervish, NA, Pajak, A, Teitelbaum, SL, Pinney, SM, Windham, GC, Kushi, LH, et al. Thyroid antagonists (perchlorate, thiocyanate, and nitrate) and childhood growth in a longitudinal study of U.S. girls. Environ Health Perspect 2016;124:542–9. https://doi.org/10.1289/ehp.1409309.Search in Google Scholar PubMed PubMed Central
129. Chen, H, Wu, L, Wang, X, Liu, Q, Ding, M, Peng, K. Perchlorate exposure and thyroid function in ammonium perchlorate workers in Yicheng, China. Int J Environ Res Publ Health 2014. https://doi.org/10.3390/ijerph110504926.Search in Google Scholar PubMed PubMed Central
130. Eguchi, A, Kunisue, T, Wu, Q, Trang, PTK, Viet, PH, Kannan, K, et al. Occurrence of Perchlorate and thiocyanate in human serum from e-waste recycling and reference sites in Vietnam: association with thyroid hormone and iodide levels. Arch Environ Contam Toxicol 2014;67:29–41. https://doi.org/10.1007/s00244-014-0021-y.Search in Google Scholar PubMed
131. Steinmaus, C, Miller, MD, Smith, AH. Perchlorate in drinking water during pregnancy and neonatal thyroid hormone levels in California. J Occup Environ Med 2010;52:1217–24. https://doi.org/10.1097/JOM.0b013e3181fd6fa7.Search in Google Scholar PubMed
132. Javidi, A, Rafiei, N, Amin, MM, Hovsepian, S, Hashemipour, M, Kelishadi, R, et al. The relationship between perchlorate in drinking water and cord blood thyroid hormones: first experience from Iran. Int J Prev Med 2015. https://doi.org/10.4103/2008-7802.151826.Search in Google Scholar PubMed PubMed Central
133. Taylor, PN, Okosieme, OE, Murphy, R, Hales, C, Chiusano, E, Maina, A, et al. Maternal perchlorate levels in women with borderline thyroid function during pregnancy and the cognitive development of their offspring: data from the controlled antenatal thyroid study. J Clin Endocrinol Metab 2014. https://doi.org/10.1210/jc.2014-1901.Search in Google Scholar PubMed
134. Schreinemachers, DM. Association between perchlorate and indirect indicators of thyroid dysfunction in NHANES 2001-2002, a cross-sectional, hypothesis-generating study. Biomark Insights 2011;6:135–46. https://doi.org/10.4137/BMI.S7985.Search in Google Scholar PubMed PubMed Central
135. Schreinemachers, DM, Ghio, AJ, Sobus, JR, Williams, MA. Perchlorate exposure is associated with oxidative stress and indicators of serum iron homeostasis among NHANES 2005-2008 subjects. Biomark Insights 2015;10:9–19. https://doi.org/10.4137/BMI.S20089.Search in Google Scholar PubMed PubMed Central
136. Liu, G, Zong, G, Dhana, K, Hu, Y, Blount, BC, Espinosa, MM, et al. Exposure to perchlorate, nitrate and thiocyanate, and prevalence of diabetes mellitus. Int J Epidemiol 2017;46:1913–23. https://doi.org/10.1093/ije/dyx188.Search in Google Scholar PubMed PubMed Central
137. Ko, WC, Liu, CL, Lee, JJ, Liu, T-P, Yang, P-S, Hsu, Y-C, et al. Negative association between serum parathyroid hormone levels and urinary perchlorate, nitrate, and thiocyanate concentrations in U.S. adults: the national health and nutrition examination survey 2005-2006. PloS One 2014;9:e115245. https://doi.org/10.1371/journal.pone.0115245.Search in Google Scholar PubMed PubMed Central
138. Shiue, I. Urinary heavy metals, phthalates, phenols, thiocyanate, parabens, pesticides, polyaromatic hydrocarbons but not arsenic or polyfluorinated compounds are associated with adult oral health: USA NHANES, 2011–2012. Environ Sci Pollut Res 2015;22:15636–45. https://doi.org/10.1007/s11356-015-4749-3.Search in Google Scholar PubMed
139. Zhang, L, Fang, C, Liu, L, Liu, X, Fan, S, Li, J, et al. A case-control study of urinary levels of iodine, perchlorate and thiocyanate and risk of papillary thyroid cancer. Environ Int 2018;120:388–93. https://doi.org/10.1016/j.envint.2018.08.024.Search in Google Scholar PubMed
140. Li, Q, Yu, YJ, Wang, FF, Chen, S-W, Yin, Y, Lin, HP, et al. Urinary perchlorate exposure and risk in women of reproductive age in a fireworks production area of China. Arch Environ Contam Toxicol 2014;67:42–9. https://doi.org/10.1007/s00244-014-0042-6.Search in Google Scholar PubMed
141. Von Oettingen, JE, Brathwaite, TD, Carpenter, C, Bonnell, R, He, X, Braverman, LE, et al. Population survey of iodine deficiency and environmental disruptors of thyroid function in Young Children in Haiti. J Clin Endocrinol Metab 2017;102:644–51. https://doi.org/10.1210/jc.2016-2630.Search in Google Scholar PubMed
142. Alomirah, HF, Al-Zenki, SF, Alaswad, MC, Alruwaih, NA, Wu, Q, Kannan, K. Widespread occurrence of perchlorate in water, foodstuffs and human urine collected from Kuwait and its contribution to human exposure. Food Addit Contam Part A Chem Anal Control Expo Risk Assess. 2016;33:1016–25. https://doi.org/10.1080/19440049.2016.1185354.Search in Google Scholar PubMed
143. Borjan, M, Marcella, S, Blount, B, Greenberg, M, Zhang, J, Murphy, E, et al. Perchlorate exposure in lactating women in an urban community in New Jersey. Sci Total Environ 2011;409:460–4. https://doi.org/10.1016/j.scitotenv.2010.10.045.Search in Google Scholar PubMed
144. Zhang, T, Wu, Q, Sun, HW, Rao, J, Kannan, K. Perchlorate and iodide in whole blood samples from infants, children, and adults in Nanchang, China. Environ Sci Technol 2010;44:6947–53. https://doi.org/10.1021/es101354g.Search in Google Scholar PubMed
145. Vega, M, Nerenberg, R, Vargas, IT. Perchlorate contamination in Chile: legacy, challenges, and potential solutions. Environ Res 2018;164:316–26. https://doi.org/10.1016/j.envres.2018.02.034.Search in Google Scholar PubMed
146. The World Health Organisation. Perchlorate in drinking water. Background document for development of WHO guidelines of drinking – water quality. Geneva, Switzerland: WHO; 2016.Search in Google Scholar
147. Suh, M, Abraham, L, Hixon, JG, Proctor, DM. The effects of perchlorate, nitrate, and thiocyanate on free thyroxine for potentially sensitive subpopulations o. The 2001-2002 and 2007-2008 National Health and Nutrition Examination Surveys. J Expo Sci Environ Epidemiol 2014;24:579–87. https://doi.org/10.1038/jes.2013.67.Search in Google Scholar PubMed
148. U.S. Environmental Protection Agency. Reductions of perchlorate in drinking water. Washington, DC: U.S. Environmental Protection Agency; 2020.Search in Google Scholar
© 2020 Przemysław Niziński et al., published by De Gruyter, Berlin/Boston
This work is licensed under the Creative Commons Attribution 4.0 International License.